I’m excited to announce my first book, PenTesting Azure Applications, is available for preorder from Amazon and No Starch’s site. This title aims to provide a reference guide for security professionals looking to assess cloud deployments. It offers practical advice for how to properly lock down subscriptions and their services, and make use of Azure’s monitoring and security features.
Don’t Believe Everything You Read
This is an archive post of content I wrote for the NTDebugging Blog on MSDN. Since the MSDN blogs are being retired, I’m transferring my posts here so they aren’t lost. The post has been back-dated to its original publication date.
Recently, I was contacted by a customer who was advised by an ISV to set a registry value under one of the sub keys in HKEY_LOCAL_MACHINE\SYSTEM\CurrentControlSet\Control\Session Manager. Let’s call it UseQuantumComputing = 1 (value name has been changed to protect the ISV). The customer wanted to know what this value actually did and no one could find any documentation explaining it. These issues often come to our team because we have access to the Windows source code. I did a bit of code review to find out what this value does. As it turns out, nowhere in Windows source code between Windows 2000 and Windows Server 2012 do we ever check for or set UseQuantumComputing.
I can think of a few reasons the ISV would suggest setting this value. Perhaps they were under the impression this did something but got confused about the value name. It’s possible they hoped making a registry change would have a placebo effect. Or, perhaps their software actually checks this value, not Windows.
The latter of these possibilities is probably the worst case scenario. An ISV should not create a registry value inside of keys used for Windows’ own internal use. Why? The first reason is that there’s no guarantee that Microsoft won’t end up coincidentally using that same value name later. This would cause a conflict between the two users of the value. Second, we have to consider the scenario where two different ISVs both decide to use the same value. That would be bad too. Lastly, there’s no guarantee that the key in use will still exist in later versions, or that it will be writable or readable by the ISV due to permission changes. In addition to all these reasons, there is the common sense issue that it is just confusing. Now the ISV’s software and uninstaller needs to look all over the registry, not just in their own keys.
On a similar note, I also recently had a case where a “Windows Tips” blog (not created, endorsed, or run by Microsoft) suggested using a registry value that was implemented in Windows but was not documented by Microsoft. It turns out this value wasn’t thoroughly tested (because it was undocumented and wasn’t intended to be used in production), and using it would cause server hangs under certain conditions. These hangs were only discovered after a large customer decided to implement the undocumented value across their enterprise.
Here are a few tips for IT Pros, developers, and users alike:
- Don’t implement random registry settings if you can’t find documentation for that setting on an official Microsoft site, like MSDN, TechNet, or support.microsoft.com(information on forums or answer boards (e.g. social.*.microsoft.com or answers.*.microsoft.com) is not official documentation). At best these unknown registry settings they will do nothing, at worst they will cause you headaches later.
- If a key/value isn’t documented, changes to it likely are not tested, and could put your machine in a state that makes it difficult or impossible to support.
- If you are a developer, keep any of your registry settings in your own key. Don’t pollute in others’ keys.
- If an ISV or Microsoft suggests you implement a setting, make sure you understand the implications of that setting.
I’ll leave you with the warning displayed in many of our KBs – it’s there for a reason!
“WARNING: If you use Registry Editor incorrectly, you may cause serious problems that may require you to reinstall your operating system. Microsoft cannot guarantee that you can solve problems that result from using Registry Editor incorrectly. Use Registry Editor at your own risk.”
Microsoft KB Registry Warning Message
-Matt
Determining the source of Bug Check 0x133 (DPC_WATCHDOG_VIOLATION) errors on Windows Server 2012
This is an archive post of content I wrote for the NTDebugging Blog on MSDN. Since the MSDN blogs are being retired, I’m transferring my posts here so they aren’t lost. The post has been back-dated to its original publication date.
What is a bug check 0x133?
Starting in Windows Server 2012, a DPC watchdog timer is enabled which will bug check a system if too much time is spent in DPC routines. This bug check was added to help identify drivers that are deadlocked or misbehaving. The bug check is of type “DPC_WATCHDOG_VIOLATION” and has a code of 0x133. (Windows 7 also included a DPC watchdog but by default, it only took action when a kernel debugger was attached to the system.) A description of DPC routines can be found at http://msdn.microsoft.com/en-us/library/windows/hardware/ff544084(v=vs.85).aspx.
The DPC_WATCHDOG_VIOLATION bug check can be triggered in two ways. First, if a single DPC exceeds a specified number of ticks, the system will stop with 0x133 with parameter 1 of the bug check set to 0. In this case, the system’s time limit for single DPC will be in parameter 3, with the number of ticks taken by this DPC in parameter 2. Alternatively, if the system exceeds a larger timeout of time spent cumulatively in all DPCs since the IRQL was raised to DPC level, the system will stop with a 0x133 with parameter 1 set to 1. Microsoft recommends that DPCs should not run longer than 100 microseconds and ISRs should not run longer than 25 microseconds, however the actual timeout values on the system are set much higher.
How to debug a 0x133 (0, …
In the case of a stop 0x133 with the first parameter set to 0, the call stack should contain the offending driver. For example, here is a debug of a 0x133 (0,…) kernel dump:
0: kd> .bugcheck
Bugcheck code 00000133
Arguments 00000000`00000000 00000000`00000283 00000000`00000282 00000000`00000000
Per MSDN, we know that this DPC has run for 0x283 ticks, when the limit was 0x282.
0: kd> k
Child-SP RetAddr Call Site
fffff803`08c18428 fffff803`098525df nt!KeBugCheckEx
fffff803`08c18430 fffff803`09723f11 nt! ??::FNODOBFM::`string'+0x13ba4
fffff803`08c184b0 fffff803`09724d98 nt!KeUpdateRunTime+0x51
fffff803`08c184e0 fffff803`09634eba nt!KeUpdateTime+0x3f9
fffff803`08c186d0 fffff803`096f24ae hal!HalpTimerClockInterrupt+0x86
fffff803`08c18700 fffff803`0963dba2 nt!KiInterruptDispatchLBControl+0x1ce
fffff803`08c18898 fffff803`096300d0 hal!HalpTscQueryCounter+0x2
fffff803`08c188a0 fffff880`04be3409 hal!HalpTimerStallExecutionProcessor+0x131
fffff803`08c18930 fffff880`011202ee ECHO!EchoEvtTimerFunc+0x7d //Here is our driver, and we can see it calls into StallExecutionProcessor
fffff803`08c18960 fffff803`097258b4 Wdf01000!FxTimer::TimerHandler+0x92
fffff803`08c189a0 fffff803`09725ed5 nt!KiProcessExpiredTimerList+0x214
fffff803`08c18ae0 fffff803`09725d88 nt!KiExpireTimerTable+0xa9
fffff803`08c18b80 fffff803`0971fe76 nt!KiTimerExpiration+0xc8
fffff803`08c18c30 fffff803`0972457a nt!KiRetireDpcList+0x1f6
fffff803`08c18da0 00000000`00000000 nt!KiIdleLoop+0x5a
Let’s view the driver’s unassembled DPC routine and see what it is doing:
0: kd> ub fffff880`04be3409
ECHO!EchoEvtTimerFunc+0x54:
fffff880`04be33e0 448b4320 mov r8d,dword ptr[rbx+20h]
fffff880`04be33e4 488b0d6d2a0000 mov rcx,qword ptr [ECHO!WdfDriverGlobals (fffff880`04be5e58)]
fffff880`04be33eb 4883631800 and qword ptr [rbx+18h],0
fffff880`04be33f0 488bd7 mov rdx,rdi
fffff880`04be33f3 ff150f260000 call qword ptr [ECHO!WdfFunctions+0x838(fffff880`04be5a08)]
fffff880`04be33f9 bbc0d40100 mov ebx,1D4C0h
fffff880`04be33fe b964000000 mov ecx,64h
fffff880`04be3403 ff15f70b0000 call qword ptr[ECHO!_imp_KeStallExecutionProcessor (fffff880`04be4000)] //It's calling KeStallExecutionProcessor with 0x64 (decimal 100) as a parameter
0: kd> u fffff880`04be3409
ECHO!EchoEvtTimerFunc+0x7d:
fffff880`04be3409 4883eb01 sub rbx,1
fffff880`04be340d 75ef jne ECHO!EchoEvtTimerFunc+0x72 (fffff880`04be33fe) //Here we can see it is jumping back to call KeStallExecutionProcessor in a loop
fffff880`04be340f 488b5c2430 mov rbx,qword ptr[rsp+30h]
fffff880`04be3414 4883c420 add rsp,20h
fffff880`04be3418 5f pop rdi
fffff880`04be3419 c3 ret
fffff880`04be341a cc int 3
fffff880`04be341b cc int 3
0: kd> !pcr
KPCR for Processor 0 at fffff80309974000:
Major 1 Minor 1
NtTib.ExceptionList: fffff80308c11000
NtTib.StackBase: fffff80308c12080
NtTib.StackLimit: 000000d70c7bf988
NtTib.SubSystemTib: fffff80309974000
NtTib.Version: 0000000009974180
NtTib.UserPointer: fffff803099747f0
NtTib.SelfTib: 000007f7ab80c000
SelfPcr: 0000000000000000
Prcb: fffff80309974180
Irql: 0000000000000000
IRR: 0000000000000000
IDR: 0000000000000000
InterruptMode: 0000000000000000
IDT: 0000000000000000
GDT: 0000000000000000
TSS: 0000000000000000
CurrentThread: fffff803099ce880
NextThread: fffffa800261cb00
IdleThread: fffff803099ce880
DpcQueue: 0xfffffa80020ce790 0xfffff880012e4e9c [Normal] NDIS!NdisReturnNetBufferLists
0xfffffa800185f118 0xfffff88000c0ca00 [Normal] ataport!AtaPortInitialize
0xfffff8030994fda0 0xfffff8030972bc30 [Normal] nt!KiBalanceSetManagerDeferredRoutine
0xfffffa8001dbc118 0xfffff88000c0ca00 [Normal] ataport!AtaPortInitialize
0xfffffa8002082300 0xfffff88001701df0 [Normal] USBPORT
The !pcr output shows us queued DPCs for this processor. If you want to see more information about DPCs and the DPC Watchdog, you could dump the PRCB listed in the !pcr output like this:
dt nt!_KPRCB fffff80309974180 Dpc*
Often the driver will be calling into a function like KeStallExecutionProcessor in a loop, as in our example debug. To resolve this problem, contact the driver vendor to request an updated driver version that spends less time in its DPC Routine.
How to troubleshoot a 0x133 (1, …
Determining the cause of a stop 0x133 with a first parameter of 1 is a bit more difficult because the problem is a result of DPCs running from multiple drivers, so the call stack is insufficient to determine the culprit. To troubleshoot this stop, first make sure that the NT Kernel Logger or Circular Kernel Context Logger ETW traces are enabled on the system. (For directions on setting this up, see http://blogs.msdn.com/b/ntdebugging/archive/2009/12/11/test.aspx.)
Once the logging is enabled and the system bug checks, dump out the list of ETW loggers using !wmitrace.strdump. Find the ID of the NT Kernel logger or the Circular logger. You can then use !wmitrace.logsave (ID) (path to ETL) to write out the ETL log to a file. Load it up with Windows Performance Analyzer and add the DPC or DPC/ISR Duration by Module, Function view (located in the Computation group) to your current analysis window:
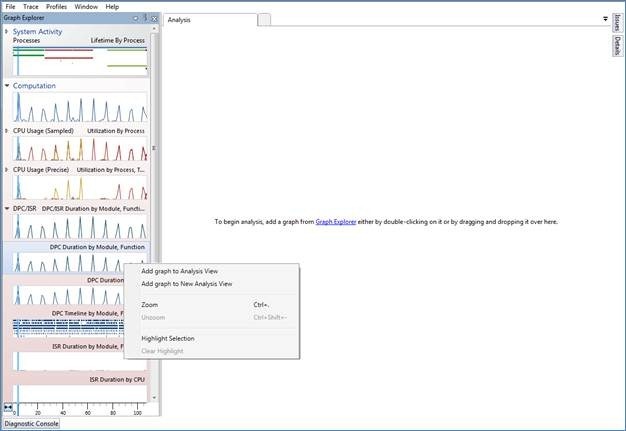
Next, make sure the table is also shown by clicking the box in the upper right of the view:
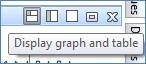
Ensure that the Address column is added on the left of the gold bar, then expand each address entry to see individual DPC enters/exits for each function. Using this data, you can determine which DPC routines took the longest by looking at the inclusive duration column, which should be added to the right of the gold bar:
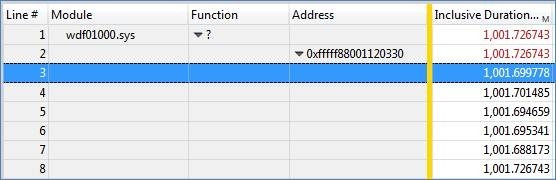
In this case, these DPCs took 1 second, which is well over the recommended maximum of 100 us. The module column (and possible the function column, if you have symbols) will show which driver is responsible for that DPC routine. Since our ECHO driver was based on WDF, that is the module named here.
For an example of doing this type of analysis in xperf, see http://blogs.msdn.com/b/ntdebugging/archive/2008/04/03/windows-performance-toolkit-xperf.aspx.
More Information
For additional information about Stop 0x133 errors, see this page on MSDN: http://msdn.microsoft.com/en-us/library/windows/hardware/jj154556(v=vs.85).aspx.
For DPC timing recommendations and for advice on capturing DPC timing information using tracelog, see http://msdn.microsoft.com/en-us/library/windows/hardware/ff545764(v=vs.85).aspx.
Guidelines for writing DPC routines can be found at http://msdn.microsoft.com/en-us/library/windows/hardware/ff546551(v=vs.85).aspx.
-Matt Burrough
How the Clipboard Works, Part 2
This is an archive post of content I wrote for the NTDebugging Blog on MSDN. Since the MSDN blogs are being retired, I’m transferring my posts here so they aren’t lost. The post has been back-dated to its original publication date.
Last time, we discussed how applications place data on the clipboard, and how to access that data using the debugger. Today, we’ll take a look at how an application can monitor the clipboard for changes. Understanding this is important, because it is a place where Windows allows 3rd-party code to “hook” into the system – so if you experience unexpected behavior with copying and pasting, a program using these hooks may be misbehaving. We’ll start by covering the hooking mechanisms for clipboard, and then review how to identify what applications, if any, are using these hooks in the debugger.
There are three ways to monitor the clipboard for changes – clipboard viewers, clipboard format listeners, and querying the clipboard sequence number. We will focus on the first two as these allow an application to register for notifications whenever the clipboard is updated. The third method simply allows an application to check and see if a change has occurred, and should not be used in a polling loop.
The Clipboard Viewer functionality has been around since Windows 2000, if not earlier. The way it works is pretty simple – an application interested in receiving clipboard change notifications calls SetClipboardViewer and passes a handle to its window. Windows then stores that handle in a per-session win32k global, and anytime the clipboard changed, windows sends a WM_DRAWCLIPBOARD message to the registered window.
Of course, multiple applications are allowed to register their windows as clipboard viewers – so how does Windows handle that? Well, if an application calls SetClipboardViewer and another clipboard viewer was already registered, Windows returns the handle value of the previous viewer’s window to the new viewer. It is then the responsibility of the new viewer to call SendMessage every time it receives a WM_DRAWCLIPBOARD to notify the next viewer in the chain. Each clipboard viewer also needs to handle the WM_CHANGECBCHAIN message, which notifies the viewers when one of the viewers in the chain was removed and specifies what the next viewer in the chain is. This allows the chain to be maintained.
An obvious problem with this design is it relies on each clipboard viewer application to behave correctly, not to terminate unexpectedly, and to generally be a good citizen. If any viewer decided not to be friendly, it could simply skip notifying the new viewer in line about an update, rendering that and all subsequent viewers impotent.
To address these problems, the Clipboard Format Listener mechanism was added in Windows Vista. This works in much the same way as the clipboard viewer functionality except in this case, Windows maintains the list of listeners, instead of depending on each application to preserve a chain.
If an application wishes to become a clipboard format listener, it calls the AddClipboardFormatListener function and passes in a handle to its window. After that, its window message handler will receive WM_CLIPBOARDUPDATE messages. When the application is ready to exit or no longer wishes to receive notifications, it can call RemoveClipboardFormatListener.
Now that we’ve covered the ways to register a viewer/listener, let’s take a look at how to identify them using the debugger. First, you’ll need to identify a process in the session you are interested in checking for clipboard monitors. It can be any win32 process in that session – we just need to use it to locate a pointer to the Window Station. In this case, I’ll use the Notepad window I used in part 1:
kd> !process 0 0 notepad.exe
PROCESS fffff980366ecb30
SessionId: 1 Cid: 0374 Peb: 7fffffd8000 ParentCid: 0814
DirBase: 1867e000 ObjectTable: fffff9803d28ef90 HandleCount: 52.
Image: notepad.exe
If you are doing this in a live kernel debug, you’ll need to change context into the process interactively (using .process /I <address> then hit g and wait for the debugger to break back in). Now DT the process address as an _EPROCESS and look for the Win32Process field:
kd> dt _EPROCESS fffff980366ecb30 Win32Process
nt!_EPROCESS
+0x258 Win32Process : 0xfffff900`c18c0ce0 Void
Now DT the Win32Process address as a win32k!tagPROCESSINFO and identify the rpwinsta value:
kd> dt win32k!tagPROCESSINFO 0xfffff900`c18c0ce0 rpwinsta
+0x258 rpwinsta : 0xfffff980`0be2af60 tagWINDOWSTATION
This is our Window Station. Dump it using dt:
kd> dt 0xfffff980`0be2af60 tagWINDOWSTATION
win32k!tagWINDOWSTATION
+0x000 dwSessionId : 1
+0x008 rpwinstaNext : (null)
+0x010 rpdeskList : 0xfffff980`0c5e2f20 tagDESKTOP
+0x018 pTerm : 0xfffff960`002f5560 tagTERMINAL
+0x020 dwWSF_Flags : 0
+0x028 spklList : 0xfffff900`c192cf80 tagKL
+0x030 ptiClipLock : (null)
+0x038 ptiDrawingClipboard : (null)
+0x040 spwndClipOpen : (null)
+0x048 spwndClipViewer : 0xfffff900`c1a4ca70 tagWND
+0x050 spwndClipOwner : 0xfffff900`c1a3ef70 tagWND
+0x058 pClipBase : 0xfffff900`c5512fa0 tagCLIP
+0x060 cNumClipFormats : 4
+0x064 iClipSerialNumber : 0x16
+0x068 iClipSequenceNumber : 0xc1
+0x070 spwndClipboardListener : 0xfffff900`c1a53440 tagWND
+0x078 pGlobalAtomTable : 0xfffff980`0bd56c70 Void
+0x080 luidEndSession : _LUID
+0x088 luidUser : _LUID
+0x090 psidUser : 0xfffff900`c402afe0 Void
Note the spwndClipViewer, spwndClipboardListener, and spwndClipOwner fields. spwndClipViewer is the most-recently-registered window in the clipboard viewer chain. Similarly, spwndClipboardListener is the most recent listener in our Clipboard Format Listener list. spwndClipOwner is the window that set the content in the clipboard.
Given the window, it is just a few steps to determine the process. This would work for spwndClipViewer, spwndClipboardListener, and spwndClipOwner. First, dt the value as a tagWND. We’ll use the spwndClipViewer for this demonstration:
kd> dt 0xfffff900`c1a4ca70 tagWND
win32k!tagWND
+0x000 head : _THRDESKHEAD
+0x028 state : 0x40020008
+0x028 bHasMeun : 0y0
+0x028 bHasVerticalScrollbar : 0y0
…
We only care about the head – so since it is at offset 0, dt the same address as a _THRDESKHEAD:
kd> dt 0xfffff900`c1a4ca70 _THRDESKHEAD
win32k!_THRDESKHEAD
+0x000 h : 0x00000000`000102ae Void
+0x008 cLockObj : 6
+0x010 pti : 0xfffff900`c4f26c20 tagTHREADINFO
+0x018 rpdesk : 0xfffff980`0c5e2f20 tagDESKTOP
+0x020 pSelf : 0xfffff900`c1a4ca70 "???"
Now, dt the address in pti as a tagTHREADINFO:
kd> dt 0xfffff900`c4f26c20 tagTHREADINFO
win32k!tagTHREADINFO
+0x000 pEThread : 0xfffff980`0ef6cb10 _ETHREAD
+0x008 RefCount : 1
+0x010 ptlW32 : (null)
+0x018 pgdiDcattr : 0x00000000`000f0d00 Void
Here, we only care about the value of pEThread, which we can pass to !thread:
kd> !thread 0xfffff980`0ef6cb10
THREAD fffff9800ef6cb10 Cid 087c.07ec Teb: 000007fffffde000 Win32Thread: fffff900c4f26c20 WAIT: (WrUserRequest) UserMode Non-Alertable
fffff9801c01efe0 SynchronizationEvent
Not impersonating
DeviceMap fffff980278a0fc0
Owning Process fffff98032e18b30 Image: viewer02.exe
Attached Process N/A Image: N/A
Wait Start TickCount 5435847 Ticks: 33 (0:00:00:00.515)
Context Switch Count 809 IdealProcessor: 0 LargeStack
UserTime 00:00:00.000
KernelTime 00:00:00.062
Win32 Start Address 0x000000013f203044
Stack Init fffff880050acdb0 Current fffff880050ac6f0
Base fffff880050ad000 Limit fffff880050a3000 Call 0
Priority 11 BasePriority 8 UnusualBoost 0 ForegroundBoost 2 IoPriority 2 PagePriority 5
Child-SP RetAddr : Args to Child : Call Site
fffff880`050ac730 fffff800`01488f32 : fffff980`0ef6cb10 fffff980`0ef6cb10 fffff980`0ef6cbd0 00000000`0000000b : nt!KiSwapContext+0x7a
fffff880`050ac870 fffff800`0148b74f : 00000000`00000021 fffff800`015b1975 00000000`00000000 00000000`6d717355 : nt!KiCommitThreadWait+0x1d2
fffff880`050ac900 fffff960`000dc8e7 : fffff900`c4f26c00 fffff880`0000000d fffff900`c40a6f01 fffff960`000dcc00 : nt!KeWaitForSingleObject+0x19f
fffff880`050ac9a0 fffff960`000dc989 : 00000000`00000000 00000000`00000000 00000000`00000001 00000000`00000000 : win32k!xxxRealSleepThread+0x257
fffff880`050aca40 fffff960`000dafc0 : fffff900`c4f26c20 fffff880`050acca0 00000000`00000001 00000000`00000000 : win32k!xxxSleepThread+0x59
fffff880`050aca70 fffff960`000db0c5 : 00000000`00000000 fffff800`000025ff 00000000`00000000 fffff980`ffffffff : win32k!xxxRealInternalGetMessage+0x7dc
fffff880`050acb50 fffff960`000dcab5 : 00000000`021503bd 00000000`021503bd fffff880`050acbc8 fffff800`01482ed3 : win32k!xxxInternalGetMessage+0x35
fffff880`050acb90 fffff800`01482ed3 : fffff980`0ef6cb10 00000000`00000000 00000000`00000020 fffff980`1c394e60 : win32k!NtUserGetMessage+0x75
fffff880`050acc20 00000000`77929e6a : 00000000`00000000 00000000`00000000 00000000`00000000 00000000`00000000 : nt!KiSystemServiceCopyEnd+0x13 (TrapFrame @ fffff880`050acc20)
00000000`002ffb18 00000000`00000000 : 00000000`00000000 00000000`00000000 00000000`00000000 00000000`00000000 : 0x77929e6a
As you can see, we have a clipboard viewer registered from process viewer02.exe. Because of viewer’s process-maintained chain architecture, it isn’t easy to see the next process in the chain. However, we can do this for clipboard listeners. Let’s look back at our window station:
kd> dt 0xfffff980`0be2af60 tagWINDOWSTATION
win32k!tagWINDOWSTATION
+0x000 dwSessionId : 1
+0x008 rpwinstaNext : (null)
+0x010 rpdeskList : 0xfffff980`0c5e2f20 tagDESKTOP
+0x018 pTerm : 0xfffff960`002f5560 tagTERMINAL
+0x020 dwWSF_Flags : 0
+0x028 spklList : 0xfffff900`c192cf80 tagKL
+0x030 ptiClipLock : (null)
+0x038 ptiDrawingClipboard : (null)
+0x040 spwndClipOpen : (null)
+0x048 spwndClipViewer : 0xfffff900`c1a4ca70 tagWND
+0x050 spwndClipOwner : 0xfffff900`c1a3ef70 tagWND
+0x058 pClipBase : 0xfffff900`c5512fa0 tagCLIP
+0x060 cNumClipFormats : 4
+0x064 iClipSerialNumber : 0x16
+0x068 iClipSequenceNumber : 0xc1
+0x070 spwndClipboardListener : 0xfffff900`c1a53440 tagWND
+0x078 pGlobalAtomTable : 0xfffff980`0bd56c70 Void
+0x080 luidEndSession : _LUID
+0x088 luidUser : _LUID
+0x090 psidUser : 0xfffff900`c402afe0 Void
If we dt the spwndClipboardListener, there is a field that shows the next listener named spwndClipboardListenerNext:
kd> dt 0xfffff900`c1a53440 tagWND spwndClipboardListenerNext
win32k!tagWND
+0x118 spwndClipboardListenerNext : 0xfffff900`c1a50080 tagWND
When you reach the last clipboard format listener’s tagWND, its spwndClipboardListenerNext value will be null:
kd> dt 0xfffff900`c1a50080 tagWND spwndClipboardListenerNext
win32k!tagWND
+0x118 spwndClipboardListenerNext : (null)
Using this window address, we can go through the same steps as above to identify this listener’s process name. As mentioned earlier, since tagWND is a kernel structure, the OS is maintaining these spwndClipboardListener/spwndClipboardListenerNext pointers, so they aren’t susceptible to the chain problems of clipboard viewers.
That wraps up our clipboard coverage. I hope you found it informative. Want to learn more about monitoring the clipboard? This MSDN article is a good resource.
-Matt Burrough
How the Clipboard Works, Part 1
This is an archive post of content I wrote for the NTDebugging Blog on MSDN. Since the MSDN blogs are being retired, I’m transferring my posts here so they aren’t lost. The post has been back-dated to its original publication date.
Recently, I had the opportunity to debug the clipboard in Windows, and I thought I’d share some of the things I learned. The clipboard is one of those parts of Windows that many of us use dozens (hundreds?) of times a day and don’t really think about. Before working on this case, I had never even considered how it works under the hood. It turns out that there’s more to it than you might think. In this first article, I’ll describe how applications store different types of data to the clipboard and how it is retrieved. My next post will describe how applications can hook the clipboard to monitor it for changes. In both, I’ll include debug notes to show you how to access the data from the debugger.
Let’s start by discussing clipboard formats. A clipboard format is used to describe what type of data is placed on the clipboard. There are a number of predefined standard formats that an application can use, such as bitmap, ANSI text, Unicode text, and TIFF. Windows also allows an application to specify its own formats. For example, a word processor may want to register a format that includes text, formatting, and images. Of course – this leads to one problem – what happens if you want to copy from your word processor and paste into Notepad, which doesn’t understand all of the formatting and pictures?
The answer is to allow multiple formats to be stored in the clipboard at one time. When I used to think of the clipboard, I thought of it as a single object (“my text” or “my image”) but in reality, the clipboard usually has my data in several different forms so when I paste, the destination program gets a format it can use.
So how does this data end up on the clipboard? Simple – an application first takes ownership of the clipboard via the OpenClipboard function. Once it has done that, it can empty the clipboard with EmptyClipboard. Finally, it is ready to place data on the clipboard using SetClipboardData. SetClipboardData takes two parameters – the first is the identifier of one of the clipboard formats we discussed above. The second is a handle to the memory containing the data in that format. The application can continue to call SetClipboardData for each of the various formats it wishes to provide going from best to worst (since the destination application will select the first format in the list it recognizes). To make things easier for the developer, Windows will automatically provide converted formats for some clipboard format types. Once the program is done, it calls CloseClipboard.
When a user hits paste, the destination application will call OpenClipboard and one of these functions to determine what data format(s) are available: IsClipboardFormatAvailable, GetPriorityClipboardFormat, or EnumClipboardFormats. Assuming the application finds a format it can use, it will then call GetClipboardData with the desired format identifier as a parameter to get a handle to the data. Finally, it will call CloseClipboard.
Now let’s take a look at how you can find what data being written to the clipboard using the debugger. (Note that all of my notes are taken from a Win7/2008 R2 system – so things might vary slightly in different versions of the OS.) Since the clipboard is part of Win32k.sys, you’ll need to use a kernel debugger. I like to use win32k!InternalSetClipboardData+0xe4 as a breakpoint. The nice thing about this offset is that it is right after we’ve populated the RDI register with data from SetClipboardData in a structure known as tagCLIP.
kd> u win32k!InternalSetClipboardData+0xe4-c L5
win32k!InternalSetClipboardData+0xd8:
fffff960`0011e278 894360 mov dword ptr [rbx+60h],eax
fffff960`0011e27b 8937 mov dword ptr [rdi],esi
fffff960`0011e27d 4c896708 mov qword ptr [rdi+8],r12
fffff960`0011e281 896f10 mov dword ptr [rdi+10h],ebp
fffff960`0011e284 ff15667e1900 call qword ptr [win32k!_imp_PsGetCurrentProcessWin32Process (fffff960`002b60f0)]
kd> dt win32k!tagCLIP
+0x000 fmt : Uint4B
+0x008 hData : Ptr64 Void
+0x010 fGlobalHandle : Int4B
Here’s what a call to SetClipboardData from Notepad looks like:
kd> k
Child-SP RetAddr Call Site
fffff880`0513a940 fffff960`0011e14f win32k!InternalSetClipboardData+0xe4
fffff880`0513ab90 fffff960`000e9312 win32k!SetClipboardData+0x57
fffff880`0513abd0 fffff800`01482ed3 win32k!NtUserSetClipboardData+0x9e
fffff880`0513ac20 00000000`7792e30a nt!KiSystemServiceCopyEnd+0x13
00000000`001dfad8 00000000`7792e494 USER32!ZwUserSetClipboardData+0xa
00000000`001dfae0 000007fe`fc5b892b USER32!SetClipboardData+0xdf
00000000`001dfb20 000007fe`fc5ba625 COMCTL32!Edit_Copy+0xdf
00000000`001dfb60 00000000`77929bd1 COMCTL32!Edit_WndProc+0xec9
00000000`001dfc00 00000000`779298da USER32!UserCallWinProcCheckWow+0x1ad
00000000`001dfcc0 00000000`ff5110bc USER32!DispatchMessageWorker+0x3b5
00000000`001dfd40 00000000`ff51133c notepad!WinMain+0x16f
00000000`001dfdc0 00000000`77a2652d notepad!DisplayNonGenuineDlgWorker+0x2da
00000000`001dfe80 00000000`77b5c521 kernel32!BaseThreadInitThunk+0xd
00000000`001dfeb0 00000000`00000000 ntdll!RtlUserThreadStart+0x1d
So here, we can dt RDI as a tagCLIP to see what was written:
kd> dt win32k!tagCLIP @rdi
+0x000 fmt : 0xd
+0x008 hData : 0x00000000`00270235 Void
+0x010 fGlobalHandle : 0n1
Fmt is the clipboard format. 0xd is 13, which indicates this data is Unicode text. We can’t just ‘du’ the value in hData, however, because this is a handle, not a direct pointer to the data. So now we need to look up the handle. To do that, we need to look at a win32k global structure – gSharedInfo:
kd> ?win32k!gSharedInfo
Evaluate expression: -7284261440224 = fffff960`002f3520
kd> dt win32k!tagSHAREDINFO fffff960`002f3520
+0x000 psi : 0xfffff900`c0980a70 tagSERVERINFO
+0x008 aheList : 0xfffff900`c0800000 _HANDLEENTRY
+0x010 HeEntrySize : 0x18
+0x018 pDispInfo : 0xfffff900`c0981e50 tagDISPLAYINFO
+0x020 ulSharedDelta : 0
+0x028 awmControl : [31] _WNDMSG
+0x218 DefWindowMsgs : _WNDMSG
+0x228 DefWindowSpecMsgs : _WNDMSG
aheList in gSharedInfo contains an array of handle entries, and the last 2 bytes of hData multiplied by the size of a handle entry the address of our handle entry:
kd> ?0x00000000`00270235 & FFFF
Evaluate expression: 565 = 00000000`00000235
kd> ??sizeof(win32k!_HANDLEENTRY)
unsigned int64 0x18
kd> ? 0xfffff900`c0800000 + (0x235*0x18)
Evaluate expression: -7693351766792 = fffff900`c08034f8
kd> dt win32k!_HANDLEENTRY fffff900`c08034f8
+0x000 phead : 0xfffff900`c0de0fb0 _HEAD
+0x008 pOwner : (null)
+0x010 bType : 0x6 ''
+0x011 bFlags : 0 ''
+0x012 wUniq : 0x27
If we look in phead at offset 14, we’ll get our data:
kd> du fffff900`c0de0fb0 + 0x14
fffff900`c0de0fc4 "Hi NTDebugging readers!"
Let’s consider one other scenario. I copied some text out of Wordpad, and a number of SetClipboardData calls were made to accommodate different formats. The Unicode format entry looks like this:
Breakpoint 0 hit
win32k!InternalSetClipboardData+0xe4:
fffff960`0011e284 ff15667e1900 call qword ptr [win32k!_imp_PsGetCurrentProcessWin32Process (fffff960`002b60f0)]
kd> dt win32k!tagCLIP @rdi
+0x000 fmt : 0xd
+0x008 hData : (null)
+0x010 fGlobalHandle : 0n0
hData is null! Why is that? It turns out that the clipboard allows an application to pass in null to SetClipboardData for a given format. This indicates that the application can provide the data in that format, but is deferring doing so until it is actually needed. Sure enough, if I paste the text into Notepad, which needs the text in Unicode, Windows sends a WM_RENDERFORMAT message to the WordPad window, and WordPad provides the data in the new format. Of course, if the application exits before populating all of its formats, Windows needs all of the formats rendered. In this case, Windows will send the WM_RENDERALLFORMATS message so other applications can use the clipboard data after the source application has exited.
That’s all for now. Next time we’ll look at how applications can monitor the clipboard for changes using two hooks. If you want to know more about using the clipboard in your code, this is a great reference.
-Matt Burrough
Part 2 of this article can be found here: How the Clipboard Works, Part 2
Debugging Backwards: Proving root cause
This is an archive post of content I wrote for the NTDebugging Blog on MSDN. Since the MSDN blogs are being retired, I’m transferring my posts here so they aren’t lost. The post has been back-dated to its original publication date.
Matt Burrough here again. On rare occasions when debugging, we’ll actually know (or strongly suspect) what the root cause of a problem is at the beginning of our analysis – but we still need to investigate to confirm our assertion. The following is a case study for an issue I worked on recently where the print spooler was hanging.
This customer had recently upgraded their print servers from Windows 2003 to Windows 2008 R2. After the upgrade, the spooler would frequently go unresponsive, and no jobs could be printed. Rebooting the server provided some relief, but the problem would reoccur as the jobs started coming in again.
One of my peers had completed an initial analysis of a user mode memory dump of the spooler process, and found that spooler seemed to be blocked waiting on PrintIsolationHost.exe. For those not familiar with recent changes to the print spooler’s design – Print Isolation Host was added in Windows 7/2008 R2 as a way to isolate print drivers from each other and from the spooler process so a crash in one driver doesn’t take down the entire printing environment. Given the large number of print drivers found on some print servers, this can be a great help for stability and availability of the spooler. See http://blogs.technet.com/b/askperf/archive/2009/10/08/windows-7-windows-server-2008-r2-print-driver-isolation.aspx if you would like more details on Print Isolation Host.
Unfortunately for my team mate, the data collected did not include dumps of Print Isolation Host, so he requested that the next time the problem happened that both spooler and PrintIsolationHost dumps would be gathered. The customer had configured his server for the “Isolated” Print Isolation Host option during troubleshooting, which places each driver in its own process. (The default is shared which places all drivers in one PrintIsolationHost.exe instance. Driver isolation is configured using the Print Management Console.)
The next morning, the newly requested data came in, and since the problem was urgent, I began looking at the new dumps immediately. This dataset included both a spoolsv.exe dump, as well as nearly two dozen PrintIsolationHost dumps! I knew from the past analysis that I should start with the PrintIsolationHost data – but where to begin? In order to triage the dumps, I wrote a small batch file to open each dump (luckily, they were sequentially numbered), write the call stacks in each thread to a file, and close the log. On every iteration, the script created a new cmd.txt file, which contained a set of commands that were passed to the debugger. This allowed me to name the debugger output files sequentially with names that matched their dump (e.g. PrintIsolationHost1.txt contained output from PrintIsolationHost1.dmp).
set x=1
:Top
echo .reload > c:\data\cmd.txt
echo .logopen c:\data\PrintIsolationHost%x%.txt >> c:\data\cmd.txt
echo ~*kc >> c:\data\cmd.txt
echo .logclose >> c:\data\cmd.txt
echo qq >> c:\data\cmd.txt
c:\debuggers\kd.exe -cf c:\data\cmd.txt -z "c:\data\PrintIsolationHost%x%.DMP"
set /A x+=1
IF %x% LEQ 23 GOTO Top
Now that I had a directory full of text files, I used my favorite differencing tool to compare the stacks in each text file. I used the first PrintIsolationHost file as a reference. It only had four stacks, and these were common to all of the other files:
. 0 Id: 40c.1c28 Suspend: 0 Teb: 000007ff`fffde000 Unfrozen
Call Site
user32!ZwUserGetMessage
user32!GetMessageW
PrintIsolationHost!ATL::CAtlExeModuleT<Host>::Run
PrintIsolationHost!Host::RunUsingConfiguration
PrintIsolationHost!wWinMain
PrintIsolationHost!__wmainCRTStartup
kernel32!BaseThreadInitThunk
ntdll!RtlUserThreadStart
1 Id: 40c.23c0 Suspend: 0 Teb: 000007ff`fffdb000 Unfrozen
Call Site
ntdll!ZwWaitForMultipleObjects
ntdll!TppWaiterpThread
kernel32!BaseThreadInitThunk
ntdll!RtlUserThreadStart
2 Id: 40c.2598 Suspend: 0 Teb: 000007ff`fffd3000 Unfrozen
Call Site
ntdll!ZwWaitForSingleObject
KERNELBASE!WaitForSingleObjectEx
PrintIsolationHost!Host::MonitorShutdown
PrintIsolationHost!Host::MonitorProc
kernel32!BaseThreadInitThunk
ntdll!RtlUserThreadStart
3 Id: 40c.820 Suspend: 0 Teb: 000007ff`fffd9000 Unfrozen
Call Site
ntdll!ZwWaitForWorkViaWorkerFactory
ntdll!TppWorkerThread
kernel32!BaseThreadInitThunk
ntdll!RtlUserThreadStart
I was able to rule out a number of other PrintIsolationHost instances that were either identical to this one (aside from the process/thread IDs and Tebs), or which just had one or two additional idle worker threads (like thread 3 above).
Things got interesting when I looked at two of the PrintIsolationHost dumps. Both had these two stacks not found in any other the other dumps (note that I do not have symbols for the 3rd-party print processor ProseWarePrintProcessor):
2 Id: 20a4.1328 Suspend: 0 Teb: 000007ff`fffda000 Unfrozen
Call Site
ntdll!ZwWaitForSingleObject
ntdll!RtlpWaitOnCriticalSection
ntdll!RtlEnterCriticalSection
ntdll!LdrLockLoaderLock
*** ERROR: Symbol file could not be found. Defaulted to export symbols for ProseWarePrintProcessor.dll -
KERNELBASE!GetModuleFileNameW
ProseWarePrintProcessor
ProseWarePrintProcessor
ProseWarePrintProcessor!ControlPrintProcessor
PrintIsolationProxy!sandbox::PrintProcessor::ControlPrintProcessor
rpcrt4!Invoke
rpcrt4!Ndr64StubWorker
rpcrt4!NdrStubCall3
ole32!CStdStubBuffer_Invoke
ole32!SyncStubInvoke
ole32!StubInvoke
ole32!CCtxComChnl::ContextInvoke
ole32!AppInvoke
ole32!ComInvokeWithLockAndIPID
ole32!ThreadInvoke
rpcrt4!DispatchToStubInCNoAvrf
rpcrt4!RPC_INTERFACE::DispatchToStubWorker
rpcrt4!RPC_INTERFACE::DispatchToStub
rpcrt4!RPC_INTERFACE::DispatchToStubWithObject
rpcrt4!LRPC_SCALL::DispatchRequest
rpcrt4!LRPC_SCALL::HandleRequest
rpcrt4!LRPC_ADDRESS::ProcessIO
rpcrt4!LrpcIoComplete
ntdll!TppAlpcpExecuteCallback
ntdll!TppWorkerThread
kernel32!BaseThreadInitThunk
ntdll!RtlUserThreadStart
6 Id: 20a4.1668 Suspend: 0 Teb: 000007ff`fffac000 Unfrozen
Call Site
ntdll!ZwWaitForSingleObject
ntdll!RtlpWaitOnCriticalSection
ntdll!RtlEnterCriticalSection
ProseWarePrintProcessor
ProseWarePrintProcessor
ProseWarePrintProcessor
ntdll!LdrpInitializeThread
ntdll!_LdrpInitialize
ntdll!LdrInitializeThunk
Interesting. Thread 6 is running the DllMain code for the ProseWarePrintProcessor DLL which holds the loader lock. It is waiting on a critical section. Meanwhile, thread 2 is waiting on the loader lock. So who holds the critical section that thread 6 wants? First, let’s find what lock thread 6 wants:
0:006> kn
# Child-SP RetAddr Call Site
00 00000000`0104eb18 00000000`777fe4e8 ntdll!ZwWaitForSingleObject+0xa
01 00000000`0104eb20 00000000`777fe3db ntdll!RtlpWaitOnCriticalSection+0xe8
02 00000000`0104ebd0 00000000`750c5d6b ntdll!RtlEnterCriticalSection+0xd1
03 00000000`0104ec00 00000000`750c6256 ProseWarePrintProcessor+0xabf
04 00000000`0104ec30 00000000`750c7015 ProseWarePrintProcessor+0xfaa
05 00000000`0104f090 00000000`777dc76c ProseWarePrintProcessor+0x1d69
06 00000000`0104f1f0 00000000`777dc42f ntdll!LdrpInitializeThread+0x17c
07 00000000`0104f2f0 00000000`777dc32e ntdll!LdrpInitialize+0x9f
08 00000000`0104f360 00000000`00000000 ntdll!LdrInitializeThunk+0xe
0:006> .frame /c /r 2
02 00000000`0104ebd0 00000000`750c5d6b ntdll!RtlEnterCriticalSection+0xd1
rax=0000000300d1001a rbx=00000000750ca330 rcx=00000000001d0000
rdx=0000000000000040 rsi=0000000000000001 rdi=0000000000000004
rip=00000000777fe3db rsp=000000000104ebd0 rbp=00000000ff000000
r8=00000000002c6a00 r9=00000000002c6a10 r10=0000000000000073
r11=0000000000000001 r12=000007fffffd6000 r13=00000000778e2660
r14=0000000000000001 r15=000000007780a280
iopl=0 nv up ei pl zr na po nc
cs=0033 ss=002b ds=002b es=002b fs=0053 gs=002b efl=00000246
ntdll!RtlEnterCriticalSection+0xd1:
00000000`777fe3db 83f801 cmp eax,1
0:006> ub 00000000`750c5d6b <----------- Let's see what gets passed to RtlEnterCriticalSection
ProseWarePrintProcessor+0xaa2:
00000000`750c5d4e f9 stc
00000000`750c5d4f ff743f33 push qword ptr [rdi+rdi+33h]
00000000`750c5d53 d2ff sar bh,cl
00000000`750c5d55 15aeb4ffff adc eax,0FFFFB4AEh
00000000`750c5d5a 85c0 test eax,eax
00000000`750c5d5c 7533 jne ProseWarePrintProcessor+0xae5 (00000000`750c5d91)
00000000`750c5d5e 488d0dcb450000 lea rcx,[ProseWarePrintProcessor+0x5084 (00000000`750ca330)] <----- Critical section is in rcx
00000000`750c5d65 ff15bdb3ffff call qword ptr [ProseWarePrintProcessor+0x1128 (00000000`750c1128)]
0:006> u ntdll!RtlEnterCriticalSection
ntdll!RtlEnterCriticalSection:
00000000`77802fc0 fff3 push rbx
00000000`77802fc2 4883ec20 sub rsp,20h
00000000`77802fc6 f00fba710800 lock btr dword ptr [rcx+8],0
00000000`77802fcc 488bd9 mov rbx,rcx <------------------ Critical section is saved in RBX. RBX isn't modified between here and our current position
00000000`77802fcf 0f83e9b1ffff jae ntdll!RtlEnterCriticalSection+0x31 (00000000`777fe1be)
00000000`77802fd5 65488b042530000000 mov rax,qword ptr gs:[30h]
00000000`77802fde 488b4848 mov rcx,qword ptr [rax+48h]
00000000`77802fe2 c7430c01000000 mov dword ptr [rbx+0Ch],1
0:006> r rbx
Last set context:
rbx=00000000750ca330 <----- This is the address of the critical section thread 6 is waiting for
Now let’s look at the threads in this process, and the held locks:
0:002> ~
# 0 Id: 20a4.180c Suspend: 0 Teb: 000007ff`fffde000 Unfrozen
1 Id: 20a4.2294 Suspend: 0 Teb: 000007ff`fffdc000 Unfrozen
. 2 Id: 20a4.1328 Suspend: 0 Teb: 000007ff`fffda000 Unfrozen
3 Id: 20a4.1c34 Suspend: 0 Teb: 000007ff`fffd8000 Unfrozen
4 Id: 20a4.5bc Suspend: 0 Teb: 000007ff`fffd4000 Unfrozen
5 Id: 20a4.3c4 Suspend: 0 Teb: 000007ff`fffae000 Unfrozen
6 Id: 20a4.1668 Suspend: 0 Teb: 000007ff`fffac000 Unfrozen
0:002> !locks
CritSec ntdll!LdrpLoaderLock+0 at 00000000778e7490
WaiterWoken No
LockCount 2
RecursionCount 1
OwningThread 1668
EntryCount 0
ContentionCount 2
*** Locked
CritSec ProseWarePrintProcessor+5084 at 00000000750ca330
WaiterWoken No
LockCount 1
RecursionCount 2
OwningThread 1328
EntryCount 0
ContentionCount 1
*** Locked
Scanned 201 critical sections
That’s not good! We can see that thread 6 indeed owns the loader lock, which thread 2 is waiting for. But thread 2 owns the ProseWarePrintProcessor lock – and thread 6 is waiting for it! This is a classic deadlock. In fact, Raymond Chen even described this on his blog. More information about LdrInitialize can be found here.
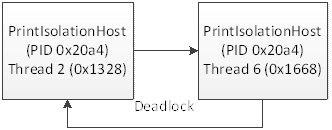
So we know that there is a deadlock in the Print Isolation Host, but why exactly does this cause spooler to hang? Here’s where we work backwards. We know that thread 6 is handling DLL initialization, but what is thread 2 doing? From the stack we can see it is handling an RPC request that called into ProseWarePrintProcessor. Let’s determine who called into this thread.
0:002> kn
# Child-SP RetAddr Call Site
00 00000000`0085dd88 00000000`777fe4e8 ntdll!ZwWaitForSingleObject+0xa
01 00000000`0085dd90 00000000`777fe3db ntdll!RtlpWaitOnCriticalSection+0xe8
02 00000000`0085de40 00000000`777db9e7 ntdll!RtlEnterCriticalSection+0xd1
03 00000000`0085de70 000007fe`fd963706 ntdll!LdrLockLoaderLock+0x6d
04 00000000`0085deb0 00000000`750c58f3 KERNELBASE!GetModuleFileNameW+0x96
05 00000000`0085df10 00000000`750c5d77 ProseWarePrintProcessor!InstallPrintProcessor+0x647
06 00000000`0085e380 00000000`750c51d9 ProseWarePrintProcessor!InstallPrintProcessor+0xacb
07 00000000`0085e3b0 000007fe`f96766b4 ProseWarePrintProcessor!ControlPrintProcessor+0x25
08 00000000`0085e3e0 000007fe`fe2f23d5 PrintIsolationProxy!sandbox::PrintProcessor::ControlPrintProcessor+0x34
09 00000000`0085e420 000007fe`fe39b68e rpcrt4!Invoke+0x65
0a 00000000`0085e470 000007fe`fe2f48d6 rpcrt4!Ndr64StubWorker+0x61b
0b 00000000`0085ea30 000007fe`fdd50883 rpcrt4!NdrStubCall3+0xb5
0c 00000000`0085ea90 000007fe`fdd50ccd ole32!CStdStubBuffer_Invoke+0x5b
0d 00000000`0085eac0 000007fe`fdd50c43 ole32!SyncStubInvoke+0x5d
0e 00000000`0085eb30 000007fe`fdc0a4f0 ole32!StubInvoke+0xdb
0f 00000000`0085ebe0 000007fe`fdd514d6 ole32!CCtxComChnl::ContextInvoke+0x190
10 00000000`0085ed70 000007fe`fdd5122b ole32!AppInvoke+0xc2
11 00000000`0085ede0 000007fe`fdd4fd6d ole32!ComInvokeWithLockAndIPID+0x52b
12 00000000`0085ef70 000007fe`fe2e50f4 ole32!ThreadInvoke+0x30d
13 00000000`0085f010 000007fe`fe2e4f56 rpcrt4!DispatchToStubInCNoAvrf+0x14
14 00000000`0085f040 000007fe`fe2e775b rpcrt4!RPC_INTERFACE::DispatchToStubWorker+0x146
15 00000000`0085f160 000007fe`fe2e769b rpcrt4!RPC_INTERFACE::DispatchToStub+0x9b
16 00000000`0085f1a0 000007fe`fe2e7632 rpcrt4!RPC_INTERFACE::DispatchToStubWithObject+0x5b
17 00000000`0085f220 000007fe`fe2e532d rpcrt4!LRPC_SCALL::DispatchRequest+0x422
18 00000000`0085f300 000007fe`fe302e7f rpcrt4!LRPC_SCALL::HandleRequest+0x20d
19 00000000`0085f430 000007fe`fe302a35 rpcrt4!LRPC_ADDRESS::ProcessIO+0x3bf
1a 00000000`0085f570 00000000`777cb68b rpcrt4!LrpcIoComplete+0xa5
1b 00000000`0085f600 00000000`777cfeff ntdll!TppAlpcpExecuteCallback+0x26b
1c 00000000`0085f690 00000000`776a652d ntdll!TppWorkerThread+0x3f8
1d 00000000`0085f990 00000000`777dc521 kernel32!BaseThreadInitThunk+0xd
1e 00000000`0085f9c0 00000000`00000000 ntdll!RtlUserThreadStart+0x1d
I know that the code in frame 19 deals with processing the RPC and has a record of the calling process’ PID and TID. In fact, from a bit of code review, I know that at this portion of the code, we get back a value that contains a ntdll!_CLIENT_ID structure at offset 8:
rpcrt4!LRPC_ADDRESS::ProcessIO+0xe6:
000007fe`fe302ba6 ff151c050a00 call qword ptr [rpcrt4!_imp_AlpcGetMessageFromCompletionList (000007fe`fe3a30c8)]
000007fe`fe302bac 4885c0 test rax,rax
000007fe`fe302baf 0f84d1020000 je rpcrt4!LRPC_ADDRESS::ProcessIO+0x3c6 (000007fe`fe302e86)
000007fe`fe302bb5 4c8bac2488000000 mov r13,qword ptr [rsp+88h]
000007fe`fe302bbd 41bc01000000 mov r12d,1
000007fe`fe302bc3 33d2 xor edx,edx
000007fe`fe302bc5 488bf8 mov rdi,rax
000007fe`fe302bc8 41bfff000000 mov r15d,0FFh
Reviewing the assembly, from ProcessIO+0xe6 to where we are now (ProcessIO+0x3bf), we don’t modify rdi again – and rdi is nonvolatile – so if we switch to that frame and check out rdi+8, we’ll know who called this thread!
0:002> .frame /c /r 19
19 00000000`0085f430 000007fe`fe302a35 rpcrt4!LRPC_ADDRESS::ProcessIO+0x3bf
rax=0000e5a47e7646ad rbx=0000000000000001 rcx=00000000750ca330
rdx=0000000000000000 rsi=00000000002b8aa0 rdi=00000000002c4a80
rip=000007fefe302e7f rsp=000000000085f430 rbp=0000000000ecff90
r8=000000000085e2d8 r9=0000000000000002 r10=0000000000000000
r11=0000000000000246 r12=0000000000ec8bf0 r13=0000000000000000
r14=0000000000000000 r15=0000000000ec8080
iopl=0 nv up ei pl zr na po nc
cs=0033 ss=002b ds=002b es=002b fs=0053 gs=002b efl=00000246
rpcrt4!LRPC_ADDRESS::ProcessIO+0x3bf:
000007fe`fe302e7f 4c8d357ad1fbff lea r14,[rpcrt4!COMMON_ResubmitListen <PERF> (rpcrt4+0x0) (000007fe`fe2c0000)]
0:002> dt _CLIENT_ID rdi+8
ntdll!_CLIENT_ID
+0x000 UniqueProcess : 0x00000000`00002694 Void
+0x008 UniqueThread : 0x00000000`000005e8 Void
0:002> ? 0x00000000`00002694
Evaluate expression: 9876 = 00000000`00002694
0:002> ? 0x00000000`000005e8
Evaluate expression: 1512 = 00000000`000005e8
So now we know that the caller was process 0x2694 and thread 0x5e8, or 9876 and 1512 in decimal, respectively. Our current process (PrintIsolationHost.exe) is PID 20a4 (see above ~ output), or 8356 decimal. So who is 9876? I happen to have a Process Listing from the dump of the data collection:
[0] 0 64 9876 spoolsv.exe Svcs: Spooler
Command Line: C:\Windows\System32\spoolsv.exe
[0] 0 64 8356 PrintIsolationHost.exe
Command Line: C:\Windows\system32\PrintIsolationHost.exe -Embedding
Okay, so I know the caller is thread 5e8 in spooler. Loading up the spooler dump, what is that thread doing?
0:000> ~~[5e8]s
ntdll!NtAlpcSendWaitReceivePort+0xa:
00000000`77801b6a c3 ret
# Child-SP RetAddr Call Site
00 00000000`0649d898 000007fe`fe2fa776 ntdll!NtAlpcSendWaitReceivePort+0xa
01 00000000`0649d8a0 000007fe`fe2f4e42 rpcrt4!LRPC_CCALL::SendReceive+0x156
02 00000000`0649d960 000007fe`fdd528c0 rpcrt4!I_RpcSendReceive+0x42
03 00000000`0649d990 000007fe`fdd5282f ole32!ThreadSendReceive+0x40
04 00000000`0649d9e0 000007fe`fdd5265b ole32!CRpcChannelBuffer::SwitchAptAndDispatchCall+0xa3
05 00000000`0649da80 000007fe`fdc0daaa ole32!CRpcChannelBuffer::SendReceive2+0x11b
06 00000000`0649dc40 000007fe`fdc0da0c ole32!CAptRpcChnl::SendReceive+0x52
07 00000000`0649dd10 000007fe`fdd5205d ole32!CCtxComChnl::SendReceive+0x68
08 00000000`0649ddc0 000007fe`fe39b949 ole32!NdrExtpProxySendReceive+0x45
09 00000000`0649ddf0 000007fe`fdd521d0 rpcrt4!NdrpClientCall3+0x2e2
0a 00000000`0649e0b0 000007fe`fdc0d8a2 ole32!ObjectStublessClient+0x11d
0b 00000000`0649e440 000007fe`fb059070 ole32!ObjectStubless+0x42
0c 00000000`0649e490 000007fe`fb057967 localspl!sandbox::PrintProcessorExecuteObserver::ControlPrintProcessor+0x10
0d 00000000`0649e4c0 000007fe`fb055e27 localspl!sandbox::PrintProcessorAdapterImpl::ControlPrintProcessor+0x27
0e 00000000`0649e4f0 000007fe`faff7392 localspl!sandbox::PrintProcessorAdapter::ControlPrintProcessor+0x1b
0f 00000000`0649e520 000007fe`faff8a0a localspl!DeleteJob+0x1ce
10 00000000`0649eae0 00000000`ff41fe25 localspl!SplSetJob+0x49e
11 00000000`0649eb80 000007fe`f9683603 spoolsv!SetJobW+0x25
12 00000000`0649ebc0 00000000`61001ce1 spoolss!SetJobW+0x1f
13 00000000`0649ec00 00000000`61001d7d Contoso!InitializePrintMonitor+0x781
14 00000000`0649ec40 000007fe`faffa674 Contoso!InitializePrintMonitor+0x81d
15 00000000`0649ec70 00000000`ff41c9c7 localspl!SplEndDocPrinter+0x214
16 00000000`0649ecd0 00000000`ff403ba6 spoolsv!EndDocPrinter+0x1f
17 00000000`0649ed00 00000000`ff3fe772 spoolsv!YEndDocPrinter+0x22
18 00000000`0649ed30 000007fe`fe2f23d5 spoolsv!RpcEndDocPrinter+0x3e
19 00000000`0649ed60 000007fe`fe39b68e rpcrt4!Invoke+0x65
1a 00000000`0649edb0 000007fe`fe2dac40 rpcrt4!Ndr64StubWorker+0x61b
1b 00000000`0649f370 000007fe`fe2e50f4 rpcrt4!NdrServerCallAll+0x40
1c 00000000`0649f3c0 000007fe`fe2e4f56 rpcrt4!DispatchToStubInCNoAvrf+0x14
1d 00000000`0649f3f0 000007fe`fe2e5679 rpcrt4!RPC_INTERFACE::DispatchToStubWorker+0x146
1e 00000000`0649f510 000007fe`fe2e532d rpcrt4!LRPC_SCALL::DispatchRequest+0x149
1f 00000000`0649f5f0 000007fe`fe302e7f rpcrt4!LRPC_SCALL::HandleRequest+0x20d
20 00000000`0649f720 000007fe`fe302a35 rpcrt4!LRPC_ADDRESS::ProcessIO+0x3bf
21 00000000`0649f860 00000000`777cb68b rpcrt4!LrpcIoComplete+0xa5
22 00000000`0649f8f0 00000000`777cfeff ntdll!TppAlpcpExecuteCallback+0x26b
23 00000000`0649f980 00000000`776a652d ntdll!TppWorkerThread+0x3f8
24 00000000`0649fc80 00000000`777dc521 kernel32!BaseThreadInitThunk+0xd
25 00000000`0649fcb0 00000000`00000000 ntdll!RtlUserThreadStart+0x1d
It’s calling into the print isolation host as we expect. It looks like it is doing that to end a print job, based on an RPC call it received. Using our same method as last time, let’s pull out the PID and TID he is responding to:
0:048> .frame /c /r 20
20 00000000`0649f720 000007fe`fe302a35 rpcrt4!LRPC_ADDRESS::ProcessIO+0x3bf
rax=000000000622986c rbx=0000000000000000 rcx=000000000622985c
rdx=000007fefe3a3c40 rsi=00000000027e5150 rdi=0000000008e6abd0
rip=000007fefe302e7f rsp=000000000649f720 rbp=0000000008e43480
r8=0000000000000010 r9=0000000000000000 r10=000007fefe2c0000
r11=0000000000000002 r12=000000000512d8c0 r13=0000000000000000
r14=0000000000000000 r15=00000000102e3710
iopl=0 nv up ei pl zr na po nc
cs=0033 ss=002b ds=002b es=002b fs=0053 gs=002b efl=00000246
rpcrt4!LRPC_ADDRESS::ProcessIO+0x3bf:
000007fe`fe302e7f 4c8d357ad1fbff lea r14,[rpcrt4!COMMON_ResubmitListen <PERF> (rpcrt4+0x0) (000007fe`fe2c0000)]
0:048> dt _CLIENT_ID rdi+8
ntdll!_CLIENT_ID
+0x000 UniqueProcess : 0x00000000`000020a4 Void
+0x008 UniqueThread : 0x00000000`00001c34 Void
Look at that, it’s doing work for a different thread back in our Print Isolation Host. Switching back to that dump, what is thread 1c34 doing?
0:002> ~~[1c34]s
ntdll!NtAlpcSendWaitReceivePort+0xa:
00000000`77801b6a c3
0:003> kn
# Child-SP RetAddr Call Site
00 00000000`00ebc098 000007fe`fe2fa776 ntdll!NtAlpcSendWaitReceivePort+0xa
01 00000000`00ebc0a0 000007fe`fe39cc74 rpcrt4!LRPC_CCALL::SendReceive+0x156
02 00000000`00ebc160 000007fe`fe39cf25 rpcrt4!NdrpClientCall3+0x244
03 00000000`00ebc420 000007fe`f9bfd878 rpcrt4!NdrClientCall3+0xf2
04 00000000`00ebc7b0 000007fe`f96845bf winspool!EndDocPrinter+0x15c
05 00000000`00ebc7f0 00000000`750c4102 spoolss!EndDocPrinter+0x2f
06 00000000`00ebc820 00000000`750c5013 ProseWarePrintProcessor+0x4102
07 00000000`00ebe620 000007fe`f9676be2 ProseWarePrintProcessor!PrintDocumentOnPrintProcessor+0xb3
08 00000000`00ebe650 000007fe`fe2f23d5 PrintIsolationProxy!sandbox::PrintProcessor::PrintDocThroughPrintProcessor+0x82
09 00000000`00ebe6b0 000007fe`fe39b68e rpcrt4!Invoke+0x65
0a 00000000`00ebe710 000007fe`fe2f48d6 rpcrt4!Ndr64StubWorker+0x61b
0b 00000000`00ebecd0 000007fe`fdd50883 rpcrt4!NdrStubCall3+0xb5
0c 00000000`00ebed30 000007fe`fdd50ccd ole32!CStdStubBuffer_Invoke+0x5b
0d 00000000`00ebed60 000007fe`fdd50c43 ole32!SyncStubInvoke+0x5d
0e 00000000`00ebedd0 000007fe`fdc0a4f0 ole32!StubInvoke+0xdb
0f 00000000`00ebee80 000007fe`fdd514d6 ole32!CCtxComChnl::ContextInvoke+0x190
10 00000000`00ebf010 000007fe`fdd5122b ole32!AppInvoke+0xc2
11 00000000`00ebf080 000007fe`fdd4fd6d ole32!ComInvokeWithLockAndIPID+0x52b
12 00000000`00ebf210 000007fe`fe2e50f4 ole32!ThreadInvoke+0x30d
13 00000000`00ebf2b0 000007fe`fe2e4f56 rpcrt4!DispatchToStubInCNoAvrf+0x14
14 00000000`00ebf2e0 000007fe`fe2e775b rpcrt4!RPC_INTERFACE::DispatchToStubWorker+0x146
15 00000000`00ebf400 000007fe`fe2e769b rpcrt4!RPC_INTERFACE::DispatchToStub+0x9b
16 00000000`00ebf440 000007fe`fe2e7632 rpcrt4!RPC_INTERFACE::DispatchToStubWithObject+0x5b
17 00000000`00ebf4c0 000007fe`fe2e532d rpcrt4!LRPC_SCALL::DispatchRequest+0x422
18 00000000`00ebf5a0 000007fe`fe302e7f rpcrt4!LRPC_SCALL::HandleRequest+0x20d
19 00000000`00ebf6d0 000007fe`fe302a35 rpcrt4!LRPC_ADDRESS::ProcessIO+0x3bf
1a 00000000`00ebf810 00000000`777cb68b rpcrt4!LrpcIoComplete+0xa5
1b 00000000`00ebf8a0 00000000`777cfeff ntdll!TppAlpcpExecuteCallback+0x26b
1c 00000000`00ebf930 00000000`776a652d ntdll!TppWorkerThread+0x3f8
1d 00000000`00ebfc30 00000000`777dc521 kernel32!BaseThreadInitThunk+0xd
1e 00000000`00ebfc60 00000000`00000000 ntdll!RtlUserThreadStart+0x1d
This thread called into spooler to end a document based on yet another RPC! To be clear, this is what we’re looking at so far:

Again, who called into this thread?
0:003> .frame /c /r 19
19 00000000`00ebf6d0 000007fe`fe302a35 rpcrt4!LRPC_ADDRESS::ProcessIO+0x3bf
rax=57633d3cd2c9c145 rbx=0000000000000000 rcx=0000000000861ff0
rdx=ffffffffff88ffe0 rsi=00000000002b8aa0 rdi=0000000000ec6bc0
rip=000007fefe302e7f rsp=0000000000ebf6d0 rbp=00000000002cdef0
r8=000000000003dbf0 r9=00000000000000fe r10=6dc0575d3d3cc4c8
r11=0000000000860020 r12=0000000000ec82b0 r13=0000000000000000
r14=0000000000000000 r15=0000000000ec8080
iopl=0 nv up ei pl zr na po nc
cs=0033 ss=002b ds=002b es=002b fs=0053 gs=002b efl=00000246
rpcrt4!LRPC_ADDRESS::ProcessIO+0x3bf:
000007fe`fe302e7f 4c8d357ad1fbff lea r14,[rpcrt4!COMMON_ResubmitListen <PERF> (rpcrt4+0x0) (000007fe`fe2c0000)]
0:003> dt _CLIENT_ID rdi+8
ntdll!_CLIENT_ID
+0x000 UniqueProcess : 0x00000000`00002694 Void
+0x008 UniqueThread : 0x00000000`000014cc Void
So another call from spooler (note the same PID as earlier) – let’s go back to spoolsv.dmp. Here is this thread:
0:048> ~~[14cc]s
ntdll!NtAlpcSendWaitReceivePort+0xa:
00000000`77801b6a c3 ret
0:049> k
Child-SP RetAddr Call Site
00000000`0351e538 000007fe`fe2fa776 ntdll!NtAlpcSendWaitReceivePort+0xa
00000000`0351e540 000007fe`fe2f4e42 rpcrt4!LRPC_CCALL::SendReceive+0x156
00000000`0351e600 000007fe`fdd528c0 rpcrt4!I_RpcSendReceive+0x42
00000000`0351e630 000007fe`fdd5282f ole32!ThreadSendReceive+0x40
00000000`0351e680 000007fe`fdd5265b ole32!CRpcChannelBuffer::SwitchAptAndDispatchCall+0xa3
00000000`0351e720 000007fe`fdc0daaa ole32!CRpcChannelBuffer::SendReceive2+0x11b
00000000`0351e8e0 000007fe`fdc0da0c ole32!CAptRpcChnl::SendReceive+0x52
00000000`0351e9b0 000007fe`fdd5205d ole32!CCtxComChnl::SendReceive+0x68
00000000`0351ea60 000007fe`fe39b949 ole32!NdrExtpProxySendReceive+0x45
00000000`0351ea90 000007fe`fdd521d0 rpcrt4!NdrpClientCall3+0x2e2
00000000`0351ed50 000007fe`fdc0d8a2 ole32!ObjectStublessClient+0x11d
00000000`0351f0e0 000007fe`fb0591ac ole32!ObjectStubless+0x42
00000000`0351f130 000007fe`fb057882 localspl!sandbox::PrintProcessorExecuteObserver::PrintDocThroughPrintProcessor+0x124
00000000`0351f1f0 000007fe`fb05601d localspl!sandbox::PrintProcessorAdapterImpl::PrintDocumentOnPrintProcessor+0x3a
00000000`0351f220 000007fe`fb013b70 localspl!sandbox::PrintProcessorAdapter::PrintDocumentOnPrintProcessor+0x9d
00000000`0351f270 000007fe`fb014c7c localspl!PrintDocumentThruPrintProcessor+0x46c
00000000`0351fa70 00000000`776a652d localspl!PortThread+0x4d0
00000000`0351fd80 00000000`777dc521 kernel32!BaseThreadInitThunk+0xd
00000000`0351fdb0 00000000`00000000 ntdll!RtlUserThreadStart+0x1d
Perfect. So now we know that localspl was printing a document, which resulted in all of these RPC calls between spooler and Print Isolation Host, and ultimately the deadlock in Print Isolation Host is holding up this thread. Just out of curiosity, are there any other threads blocked on this wait chain?
0:049> !locks
CritSec +5a00060 at 0000000005a00060
WaiterWoken No
LockCount 105
RecursionCount 1
OwningThread 5e8
EntryCount 0
ContentionCount 84
*** Locked
Scanned 14148 critical sections
Yes there are. Thread 5e8, which we looked at earlier, is holding a lock that 104 other threads are waiting for! This dump had 177 threads, so we know now that thread 5e8, 14cc, and 104 others in spooler are all hung on this deadlock. With about 60% of all the threads in spooler hung, the deadlock in ProseWarePrintProcessor is clearly the cause of our issue. Here’s the final wait chain diagram:
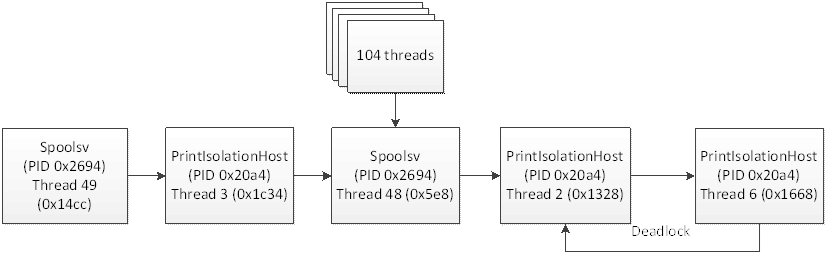
To resolve the issue, ProseWarePrintProcessor needs to avoid calling GetModuleFileName while its DllMain routine is still running, since the former requires, and the latter holds, the Loader Lock.
Test Mode, and other desktop watermarks
This is an archive post of content I wrote for the NTDebugging Blog on MSDN. Since the MSDN blogs are being retired, I’m transferring my posts here so they aren’t lost. The post has been back-dated to its original publication date.
Hi all, Matt here again. One of our team’s main functions is to work with our development teams to create hotfixes when customers run into issues that can only be resolved through a code change. The developers will often prepare a private hotfix that either tests the proposed change, or adds additional instrumentation to help pinpoint the issue. The private hotfix is sent to the customer reporting the problem so they can confirm that it does indeed correct (or identify) the flaw.
When testing a private hotfix, customers frequently ask, why does my desktop now show a message on the lower right corner of the desktop, and what does it mean? The message reads “For testing purposes only”, and looks like this:
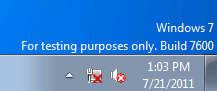
Often, users are concerned that his message means that they aren’t allowed to use the server in production, or maybe that it is now “unsupported.” These aren’t the case! Since this message appears as a result of installing a fix during the course of a Microsoft Support case, the servers are, by definition, being supported.
The purpose of this message is simply to remind users that code that Microsoft Support has asked them to test has been installed on the system, and this code may not have yet undergone the full suite of quality assurance testing that fixes that are made public do.
For comparison, let’s look at some of the other watermarks you may find in the lower corner of the desktop – as these can often be confused for the above message, and may explain some of the fears around these messages.
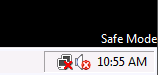
First up is the old trusty text you see when a box is booted into ‘Safe Mode’. I’m sure every IT Pro has seen this at one time or another, so I won’t go into detail, but rest assured, the testing purposes text is completely unrelated to booting in safe mode or having a subset of services running.
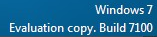
Next up is our ‘Evaluation copy’ watermark. This message is shown on the desktops of copies of Windows that have a “time bomb” (ones that will cease to function after a certain date.) This message is typically seen on beta versions of Windows which are designed to stop functioning sometime after the desired beta testing period ends.
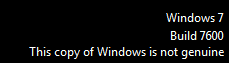
Third, we have our Windows is not genuine message. This is shown if, for example, a copy of Windows is not activated during the grace period after the installation process, or if a number of hardware changes have been made and Windows needs to be re-activated. This has nothing to do with the ‘testing purposes’ message. See http://technet.microsoft.com/en-us/library/dd979803.aspx for more information about this message.

Fourth, we have the general Windows build stamp. This is enabled via the registry using the PaintDesktopVersion DWORD (http://technet.microsoft.com/en-us/library/cc782257(WS.10).aspx). Some administrators like to enable this option so they always know what version of Windows they are using, sort of like a mini-bginfo. Unlike all of the others, this message does not indicate anything else about a server’s state.
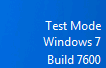
And finally, we have ‘Test Mode’. This is actually somewhat related to the testing purposes message. This ‘Test Mode’ text is shown when test signing is enabled on a PC. This is done by running “bcdedit /set testsigning on” from an UAC-elevated command prompt. Test signing is used to allow developers to load drivers they are still working on that have not yet been code signed with an official certificate. This is actually one of the steps we need to do when loading our test fixes. For more information on Test Signing, see http://msdn.microsoft.com/en-us/library/ff553484%28v=VS.85%29.aspx.
So now that you know what causes these various watermarks to appear, perhaps you’re wondering how to make the “For testing purposes only” message disappear. This is a question we are frequently asked. While you are running a test hotfix, there is no way to disable this message. Your only option is to remove the test hotfix from your system. This is something your engineer will ask you to do before you install the final, public version of a hotfix. You can easily identify and uninstall test hotfixes by going into Control Panel, Programs and Features, View Installed Updates, then look for hotfixes with the words FOR TESTING PURPOSES ONLY in their name, like the one shown in the image below. You may also notice that the KB number listed for these fixes is often a place holder, and not a real KB article ID.
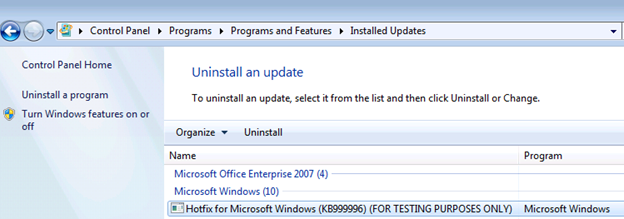
If the ‘For testing purposes only’ message is still displayed even after uninstalling the test hotfix, there is one more place to check. If a system has the Microsoft Test Root Authority certificate installed into its Trusted Root Certification Authorities store, the text will be displayed. We use this certificate to allow a PC to run test code that has been signed by our development team, but not yet fully tested and signed off with the official Microsoft certificate. To remove this certificate from your system, go to Start -> Run, and enter certmgr.msc and hit enter. In the Certificate Manager MMC, browse to Trusted Root Certification Authorities, then into Certificates. You should see one labeled Microsoft Test Root Authority, as shown below. This will need to be deleted and the system rebooted to remove the testing purposes message. Don’t do this if you still have a test hotfix installed though, as it would prevent that binary from continuing to function and may mean you can no longer boot in to Normal or Safe mode.
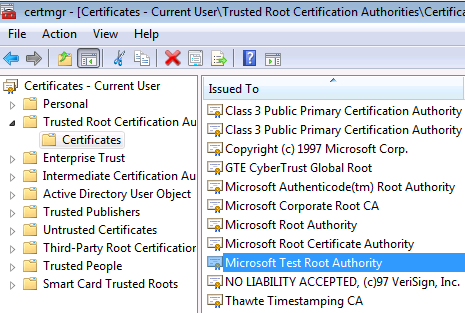
If you reboot and find that ‘Text Mode’ has replaced the ‘For testing purposes only’ text, you’ll need to launch a command prompt with administrative privileges, then run “bcdedit /set testsigning off” and reboot. You can always check if test signing is enabled by running “bcdedit /enum” and looking for this line:
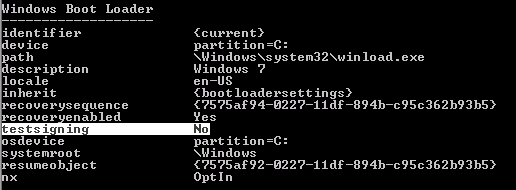
That’s all for today. Hopefully this post helped clear up any confusion about our different desktop watermarks.
Control Panel Policy and the Missing Icon
This is an archive post of content I wrote for the NTDebugging Blog on MSDN. Since the MSDN blogs are being retired, I’m transferring my posts here so they aren’t lost. The post has been back-dated to its original publication date.
A customer recently reported a rather peculiar problem. They were working on a roll out of Windows 7, and one of the policies they employed on their domain was the “Show only specified control panel applets” setting. As its name implies, this policy allows an administrator to specify the names of any Control Panel icons they want their users to see, and all others are not shown. This company chose to allow a few of the Control Panel items included with Windows as well as the Mail icon that is added when Microsoft Office (specifically Outlook) is installed, which allows users to configure their Outlook profiles.
The problem the customer noticed was that, with the policy was in place, the first time a user opened the Control Panel on a computer, all of the allowed icons appeared except for Mail. If the user closed Control Panel and reopened it, the icon suddenly appeared. In fact, from that point on, the Mail icon would appear for that user on that computer. However, if that user logged on to a different computer, or a different user logged in to the first user’s computer, the icon was missing until Control Panel was opened for a second time.
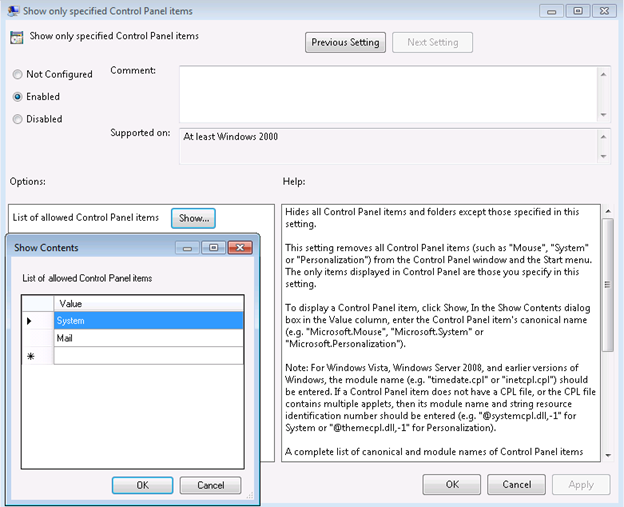
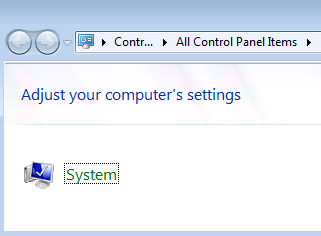
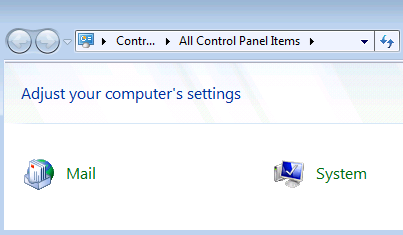
Control Panel isn’t something we get many cases on, so I had to figure out how it worked, and what would cause it to fail only the first time. One of the first things I discovered as I debugged the issue was that the Control Panel is actually loaded in two passes – a “fast” pass and a “slow” pass. This is done so icons we know will be loaded quickly can be displayed first so a user doesn’t get stuck with an empty window waiting for some of the slower icons to load. What makes an icon fast or slow? It turns out shell maintains a cache of Control Panel items, so it can display them quickly without having to query the actual item for its name, description, icon, etc. If we have to ask the item for its information, that will take longer, so it is loaded during the second, slow phase:
shell32!CControlPanelDataWorkItem::_LoadSlowData
shell32!CControlPanelDataWorkItem::DoWork
shell32!CFrameTask::InternalResumeRT
shell32!CRunnableTask::Run
shell32!CShellTask::TT_Run
shell32!CShellTaskThread::ThreadProc
shell32!CShellTaskThread::s_ThreadProc
shlwapi!ExecuteWorkItemThreadProc
ntdll!RtlpTpWorkCallback
ntdll!TppWorkerThread
kernel32!BaseThreadInitThunk
ntdll!__RtlUserThreadStart
ntdll!_RtlUserThreadStart
Luckily for these icons, they won’t stay relegated to slow loading status forever. During the slow loading phase, one of the things we do is add the item’s information to the Control Panel cache, so we can load it during the fast phase in the future.
shell32!CPLD_AddControlToReg
shell32!CControlPanelEnum::_NextNonCachedCpl
shell32!CControlPanelEnum::Next
shell32!CRegFolderEnum::Next
shell32!CControlPanelAppletList::_AddAppletsToCategories
shell32!CControlPanelAppletList::LoadSlowApplets
shell32!CControlPanelDataWorkItem::_LoadSlowData
shell32!CControlPanelDataWorkItem::DoWork
shell32!CFrameTask::InternalResumeRT
shell32!CRunnableTask::Run
shell32!CShellTask::TT_Run
shell32!CShellTaskThread::ThreadProc
shell32!CShellTaskThread::s_ThreadProc
shlwapi!ExecuteWorkItemThreadProc
ntdll!RtlpTpWorkCallback
ntdll!TppWorkerThread
kernel32!BaseThreadInitThunk
ntdll!__RtlUserThreadStart
ntdll!_RtlUserThreadStart
This explains why the item was being loaded on subsequent opens of Control Panel – the item was added to the user’s cache on the first run, so the next time, it was loaded during the fast pass:
shell32!CControlPanelEnum::_NextCachedCpl
shell32!CControlPanelEnum::Next
shell32!CRegFolderEnum::Next
shell32!CControlPanelAppletList::_AddAppletsToCategories
shell32!CControlPanelAppletList::EnsureLoaded
shell32!CControlPanelDataWorkItem::_LoadFastData
shell32!CControlPanelDataWorkItem::DoWork
shell32!CFrameTask::InternalResumeRT
shell32!CRunnableTask::Run
shell32!CShellTask::TT_Run
shell32!CShellTaskThread::ThreadProc
shell32!CShellTaskThread::s_ThreadProc
shlwapi!ExecuteWorkItemThreadProc
ntdll!RtlpTpWorkCallback
ntdll!TppWorkerThread
kernel32!BaseThreadInitThunk
ntdll!__RtlUserThreadStart
ntdll!_RtlUserThreadStart
But that only answers half the question – while we now know why the icon appears every other time, we still don’t know why it doesn’t show up the first time. To figure this out, I debugged a system with the “Show only specified control panel applets” policy applied and one without the policy set, both as they opened Control Panel for the first time, and compared where the return codes from the functions diverged.
Starting with CControlPanelEnum::_NextNonCachedCpl, in the non-working case, we can see that it returns 0:
0:003> bp shell32!CControlPanelEnum::_NextNonCachedCpl
0:003> g
Breakpoint 0 hit
eax=14c5fa8c ebx=14c5fa7c ecx=11224630 edx=00000000 esi=11224630 edi=14c5fa8c
eip=76977e11 esp=14c5f9b4 ebp=14c5f9c8 iopl=0 nv up ei pl zr na pe nc
cs=001b ss=0023 ds=0023 es=0023 fs=003b gs=0000 efl=00000246
shell32!CControlPanelEnum::_NextNonCachedCpl:
76977e11 8bff mov edi,edi
0:020> k
ChildEBP RetAddr
14c5f9b0 76977dff shell32!CControlPanelEnum::_NextNonCachedCpl
14c5f9c8 768a854d shell32!CControlPanelEnum::Next+0x5a
14c5fa18 769710d2 shell32!CRegFolderEnum::Next+0x22e
14c5fa94 769783e7 shell32!CControlPanelAppletList::_AddAppletsToCategories+0x35
14c5fac4 76978353 shell32!CControlPanelAppletList::LoadSlowApplets+0x68
14c5faec 76974db4 shell32!CControlPanelDataWorkItem::_LoadSlowData+0x2e
14c5fb00 769e06ad shell32!CControlPanelDataWorkItem::DoWork+0x4b
14c5fb18 768d83a6 shell32!CFrameTask::InternalResumeRT+0x14
14c5fb38 7691639b shell32!CRunnableTask::Run+0xce
14c5fb54 76918c1f shell32!CShellTask::TT_Run+0x167
14c5fb9c 76918d53 shell32!CShellTaskThread::ThreadProc+0xa3
14c5fba4 760cb2b1 shell32!CShellTaskThread::s_ThreadProc+0x1b
14c5fbb4 77c8d877 shlwapi!ExecuteWorkItemThreadProc+0xe
14c5fc28 77c90842 ntdll!RtlpTpWorkCallback+0x11d [d:\w7rtm\minkernel\threadpool\compat\worker.c @ 284]
14c5fd80 00000000 ntdll!TppWorkerThread+0x572
0:020> gu * <------------- This steps out to the instruction after the call to CControlPanelEnum::_NextNonCachedCpl in CControlPanelEnum::Next.
ModLoad: 35c70000 35c8b000 c:\progra~1\common~1\system\msmapi\1033\mlcfg32.cpl
eax=00000000 ebx=14c5fa7c ecx=bea347f1 edx=006d2904 esi=11224630 edi=14c5fa8c
eip=76977dff esp=14c5f9bc ebp=14c5f9c8 iopl=0 nv up ei pl zr na pe nc
cs=001b ss=0023 ds=0023 es=0023 fs=003b gs=0000 efl=00000246
shell32!CControlPanelEnum::Next+0x5a:
76977dff 84c0 test al,al
But on the machine without the policy set, it returns 1:
eax=0c83fc34 ebx=0c83fc24 ecx=04ab1060 edx=00000000 esi=04ab1060 edi=0c83fc34
eip=76977e11 esp=0c83fb5c ebp=0c83fb70 iopl=0 nv up ei pl zr na pe nc
cs=001b ss=0023 ds=0023 es=0023 fs=003b gs=0000 efl=00000246
shell32!CControlPanelEnum::_NextNonCachedCpl:
76977e11 8bff mov edi,edi
0:012> k
ChildEBP RetAddr
0c83fb58 76977dff shell32!CControlPanelEnum::_NextNonCachedCpl
0c83fb70 768a854d shell32!CControlPanelEnum::Next+0x5a
0c83fbc0 769710d2 shell32!CRegFolderEnum::Next+0x22e
0c83fc3c 769783e7 shell32!CControlPanelAppletList::_AddAppletsToCategories+0x35
0c83fc6c 76978353 shell32!CControlPanelAppletList::LoadSlowApplets+0x68
0c83fc94 76974db4 shell32!CControlPanelDataWorkItem::_LoadSlowData+0x2e
0c83fca8 769e06ad shell32!CControlPanelDataWorkItem::DoWork+0x4b
0c83fcc0 768d83a6 shell32!CFrameTask::InternalResumeRT+0x14
0c83fce0 7691639b shell32!CRunnableTask::Run+0xce
0c83fcfc 76918c1f shell32!CShellTask::TT_Run+0x167
0c83fd44 76918d53 shell32!CShellTaskThread::ThreadProc+0xa3
0c83fd4c 760cb2b1 shell32!CShellTaskThread::s_ThreadProc+0x1b
0c83fd5c 77c8d877 shlwapi!ExecuteWorkItemThreadProc+0xe
0c83fdd0 77c90842 ntdll!RtlpTpWorkCallback+0x11d
0c83ff30 778b3c45 ntdll!TppWorkerThread+0x572
0c83ff3c 77cc37f5 kernel32!BaseThreadInitThunk+0xe
0c83ff7c 77cc37c8 ntdll!__RtlUserThreadStart+0x70
0c83ff94 00000000 ntdll!_RtlUserThreadStart+0x1b
0:012> gu
ModLoad: 35c70000 35c8b000 c:\progra~1\common~1\system\msmapi\1033\mlcfg32.cpl
eax=00000001 ebx=0c83fc24 ecx=bea347f1 edx=00002bba esi=04ab1060 edi=0c83fc34
eip=76977dff esp=0c83fb64 ebp=0c83fb70 iopl=0 nv up ei pl zr na pe nc
cs=001b ss=0023 ds=0023 es=0023 fs=003b gs=0000 efl=00000246
shell32!CControlPanelEnum::Next+0x5a:
76977dff 84c0 test al,al
So we know something in this function is causing us to return FALSE in the failing case and TRUE in the working case. An easy way to see where the return result of function calls is the wt (watch trace) debugger command with the -oR switch to see the return values. I set the level (-l) to 1 so I’d only see functions directly called from CControlPanelEnum::_NextNonCachedCpl. Here were the results.
Failing case:
eax=14c5fa8c ebx=14c5fa7c ecx=11224630 edx=00000000 esi=11224630 edi=14c5fa8c
eip=76977e11 esp=14c5f9b4 ebp=14c5f9c8 iopl=0 nv up ei pl zr na pe nc
cs=001b ss=0023 ds=0023 es=0023 fs=003b gs=0000 efl=00000246
shell32!CControlPanelEnum::_NextNonCachedCpl:
76977e11 8bff mov edi,edi
0:020> wt -oR -l 1
Tracing shell32!CControlPanelEnum::_NextNonCachedCpl to return address 76977dff
31 0 [ 0] shell32!CControlPanelEnum::_NextNonCachedCpl
10 0 [ 1] shell32!DSA_GetItemPtr
17 0 [ 1] comctl32!DSA_GetItemPtr eax = 1123aa78
48 27 [ 0] shell32!CControlPanelEnum::_NextNonCachedCpl
ModLoad: 35c70000 35c8b000 c:\progra~1\common~1\system\msmapi\1033\mlcfg32.cpl
34 0 [ 1] shell32!CPLD_InitModule eax = 1
55 61 [ 0] shell32!CControlPanelEnum::_NextNonCachedCpl
15 0 [ 1] shell32!CDPA_Base<RESULT_FOLDER_ITEM,CTContainer_PolicyUnOwned<RESULT_FOLDER_ITEM> >::AppendPtr eax = 0
59 76 [ 0] shell32!CControlPanelEnum::_NextNonCachedCpl
14 0 [ 1] shell32!CPL_AddModuleReference eax = 0
61 90 [ 0] shell32!CControlPanelEnum::_NextNonCachedCpl
21 0 [ 1] shell32!CPL_ReleaseModuleReference eax = 0
76 111 [ 0] shell32!CControlPanelEnum::_NextNonCachedCpl
128 0 [ 1] shell32!CPLD_AddControlToReg eax = 1
78 239 [ 0] shell32!CControlPanelEnum::_NextNonCachedCpl
12 0 [ 1] shell32!FindCPLModuleInList eax = c666998
84 251 [ 0] shell32!CControlPanelEnum::_NextNonCachedCpl
10 0 [ 1] shell32!DSA_GetItemPtr
17 0 [ 1] comctl32!DSA_GetItemPtr eax = c5e7410
91 278 [ 0] shell32!CControlPanelEnum::_NextNonCachedCpl
90 0 [ 1] shell32!CControlPanelEnum::_CanEnumerateApplet eax = 0
97 368 [ 0] shell32!CControlPanelEnum::_NextNonCachedCpl
21 0 [ 1] shell32!CPL_ReleaseModuleReference eax = 0
108 389 [ 0] shell32!CControlPanelEnum::_NextNonCachedCpl
143 0 [ 1] shell32!CPLD_FlushRegModules eax = 0
116 532 [ 0] shell32!CControlPanelEnum::_NextNonCachedCpl
3 0 [ 1] shell32!__security_check_cookie eax = 0
118 535 [ 0] shell32!CControlPanelEnum::_NextNonCachedCpl
Working case:
eax=0c83fc34 ebx=0c83fc24 ecx=04ab1060 edx=00000000 esi=04ab1060 edi=0c83fc34
eip=76977e11 esp=0c83fb5c ebp=0c83fb70 iopl=0 nv up ei pl zr na pe nc
cs=001b ss=0023 ds=0023 es=0023 fs=003b gs=0000 efl=00000246
shell32!CControlPanelEnum::_NextNonCachedCpl:
76977e11 8bff mov edi,edi
0:012> wt -oR -l 1
Tracing shell32!CControlPanelEnum::_NextNonCachedCpl to return address 76977dff
31 0 [ 0] shell32!CControlPanelEnum::_NextNonCachedCpl
10 0 [ 1] shell32!DSA_GetItemPtr
17 0 [ 1] comctl32!DSA_GetItemPtr eax = 4a732c8
48 27 [ 0] shell32!CControlPanelEnum::_NextNonCachedCpl
ModLoad: 35c70000 35c8b000 c:\progra~1\common~1\system\msmapi\1033\mlcfg32.cpl
34 0 [ 1] shell32!CPLD_InitModule eax = 1
55 61 [ 0] shell32!CControlPanelEnum::_NextNonCachedCpl
15 0 [ 1] shell32!CDPA_Base<RESULT_FOLDER_ITEM,CTContainer_PolicyUnOwned<RESULT_FOLDER_ITEM> >::AppendPtr eax = 0
59 76 [ 0] shell32!CControlPanelEnum::_NextNonCachedCpl
14 0 [ 1] shell32!CPL_AddModuleReference eax = 0
61 90 [ 0] shell32!CControlPanelEnum::_NextNonCachedCpl
21 0 [ 1] shell32!CPL_ReleaseModuleReference eax = 0
76 111 [ 0] shell32!CControlPanelEnum::_NextNonCachedCpl
128 0 [ 1] shell32!CPLD_AddControlToReg eax = 1
78 239 [ 0] shell32!CControlPanelEnum::_NextNonCachedCpl
12 0 [ 1] shell32!FindCPLModuleInList eax = 2ff3968
84 251 [ 0] shell32!CControlPanelEnum::_NextNonCachedCpl
10 0 [ 1] shell32!DSA_GetItemPtr
17 0 [ 1] comctl32!DSA_GetItemPtr eax = 4aa81a8
91 278 [ 0] shell32!CControlPanelEnum::_NextNonCachedCpl
111 0 [ 1] shell32!CControlPanelEnum::_CanEnumerateApplet eax = 1
104 389 [ 0] shell32!CControlPanelEnum::_NextNonCachedCpl
21 0 [ 1] shell32!CPL_ReleaseModuleReference eax = 0
112 410 [ 0] shell32!CControlPanelEnum::_NextNonCachedCpl
3 0 [ 1] shell32!__security_check_cookie eax = 1
114 413 [ 0] shell32!CControlPanelEnum::_NextNonCachedCpl
Here, we can see that the function CControlPanelEnum::_CanEnumerateApplet returns true in the working case, but false in the failing case. This is what is causing the return value from _NextNonCachedCpl to differ, which is ultimately what causes the icon to load/not load. So let’s take a look at _CanEnumerateApplet. Using wt inside this function showed something rather interesting:
Failing:
eax=0c5e7410 ebx=769298fd ecx=11224630 edx=00000000 esi=11224630 edi=0c666998
eip=7693a72f esp=14c5f980 ebp=14c5f9b0 iopl=0 nv up ei pl nz na po nc
cs=001b ss=0023 ds=0023 es=0023 fs=003b gs=0000 efl=00000202
shell32!CControlPanelEnum::_CanEnumerateApplet:
7693a72f 8bff mov edi,edi
0:020> wt -oR -l 1
Tracing shell32!CControlPanelEnum::_CanEnumerateApplet to return address 7693a68e
27 0 [ 0] shell32!CControlPanelEnum::_CanEnumerateApplet
737 0 [ 1] shlwapi!PathFindFileNameW eax = c6669f8
40 737 [ 0] shell32!CControlPanelEnum::_CanEnumerateApplet
59 0 [ 1] shell32!IDControlCreate eax = 0
44 796 [ 0] shell32!CControlPanelEnum::_CanEnumerateApplet
43 0 [ 1] shlwapi!SHWindowsPolicy eax = 1
53 839 [ 0] shell32!CControlPanelEnum::_CanEnumerateApplet
2 0 [ 1] shell32!CRegFolder::QueryInterface
5 0 [ 1] shell32!CKnownFoldersFolder::QueryInterface
12 0 [ 1] shell32!CAggregatedUnknown::QueryInterface eax = 0
71 858 [ 0] shell32!CControlPanelEnum::_CanEnumerateApplet
48 0 [ 1] shell32!CRegFolder::GetDetailsEx eax = ffffffff`80070002
77 906 [ 0] shell32!CControlPanelEnum::_CanEnumerateApplet
24 0 [ 1] shell32!CRegFolder::Release eax = 3
79 930 [ 0] shell32!CControlPanelEnum::_CanEnumerateApplet
6 0 [ 1] shell32!ATL::CComVariant::Clear eax = 0
88 936 [ 0] shell32!CControlPanelEnum::_CanEnumerateApplet
3 0 [ 1] shell32!__security_check_cookie eax = 0
90 939 [ 0] shell32!CControlPanelEnum::_CanEnumerateApplet
Working:
0:012> wt -oR -l 1
Tracing shell32!CControlPanelEnum::_CanEnumerateApplet to return address 7693a68e
27 0 [ 0] shell32!CControlPanelEnum::_CanEnumerateApplet
737 0 [ 1] shlwapi!PathFindFileNameW eax = 2ff39c8
40 737 [ 0] shell32!CControlPanelEnum::_CanEnumerateApplet
59 0 [ 1] shell32!IDControlCreate eax = 0
44 796 [ 0] shell32!CControlPanelEnum::_CanEnumerateApplet
43 0 [ 1] shlwapi!SHWindowsPolicy eax = 1
53 839 [ 0] shell32!CControlPanelEnum::_CanEnumerateApplet
2 0 [ 1] shell32!CRegFolder::QueryInterface
5 0 [ 1] shell32!CKnownFoldersFolder::QueryInterface
12 0 [ 1] shell32!CAggregatedUnknown::QueryInterface eax = 0
71 858 [ 0] shell32!CControlPanelEnum::_CanEnumerateApplet
48 0 [ 1] shell32!CRegFolder::GetDetailsEx eax = 0
77 906 [ 0] shell32!CControlPanelEnum::_CanEnumerateApplet
28 0 [ 1] shell32!CPL_DoesPolicyAllow eax = 1
82 934 [ 0] shell32!CControlPanelEnum::_CanEnumerateApplet
24 0 [ 1] shell32!CRegFolder::Release eax = 3
84 958 [ 0] shell32!CControlPanelEnum::_CanEnumerateApplet
6 0 [ 1] shell32!ATL::CComVariant::Clear eax = 0
98 964 [ 0] shell32!CControlPanelEnum::_CanEnumerateApplet
358 0 [ 1] shell32!CControlPanelFolder::GetCustomAttributes eax = 0
109 1322 [ 0] shell32!CControlPanelEnum::_CanEnumerateApplet
3 0 [ 1] shell32!__security_check_cookie eax = 1
111 1325 [ 0] shell32!CControlPanelEnum::_CanEnumerateApplet
Looking at the two, we can see that they both call GetDetailsEx, but in the failing case we seem to get an error result: 80070002, and in the working case we get 0, and then proceed to call CPL_DoesPolicyAllow. CPL_DoesPolicyAllow is not called on the machine with the policy applied. A quick code review of CPL_DoesPolicyAllow showed that this function checks if a policy prevents or allows an item from being displayed. So if we can just get to that function, the icon will load. Now we need to know why GetDetailsEx fails, preventing us from calling CPL_DoesPolicyAllow. If we look up the error code, it is pretty generic:
0:020> !error 80070002
Error code: (HRESULT) 0x80070002 (2147942402) - The system cannot find the file specified.
Next I stepped through and into GetDetailsEx. I’ll save you the tedious steps and output, since the procedure is similar to what I’ve already shown above. Anyway, I was able to trace the error 2 as being returned by a call to the registry a few functions deep into GetDetailsEx:
kernel32!RegOpenKeyExW
shell32!CControlPanelFolder::_GetExtPropRegKey
shell32!CControlPanelFolder::_InitExtPropRegValNameCache
shell32!CControlPanelFolder::_PropertyFromPidl
shell32!CControlPanelFolder::GetDetailsEx
shell32!CRegFolder::GetDetailsEx
shell32!CControlPanelEnum::_CanEnumerateApplet
shell32!CControlPanelEnum::_NextNonCachedCpl
This is good news, because it means we can find out what we’re looking for that we aren’t finding. First I stepped out to get back to CControlPanelFolder::_InitExtPropRegValNameCache. Sure enough, eax was 2, which is our ‘cannot find the file’ error.
0:020> gu
eax=00000002 ebx=00000000 ecx=778ac2da edx=00000002 esi=14c5eff0 edi=00000000
eip=76975ead esp=14c5ed40 ebp=14c5efc4 iopl=0 nv up ei pl zr na pe nc
cs=001b ss=0023 ds=0023 es=0023 fs=003b gs=0000 efl=00000246
shell32!CControlPanelFolder::_GetExtPropRegKey+0xb1:
76975ead 50 push eax
0:020> !error 2
Error code: (Win32) 0x2 (2) - The system cannot find the file specified
Since we know that RegOpenKeyEx takes the subkey it is looking for as the second parameter, let’s look back at the assembly before the call to see what we passed in:
0:020> ub @eip
shell32!CControlPanelFolder::_GetExtPropRegKey+0x98:
76975e94 7c1f jl shell32!CControlPanelFolder::_GetExtPropRegKey+0xb9 (76975eb5)
76975e96 56 push esi
76975e97 6a01 push 1
76975e99 53 push ebx
76975e9a 8d8590fdffff lea eax,[ebp-270h]
76975ea0 50 push eax
76975ea1 ffb588fdffff push dword ptr [ebp-278h]
76975ea7 ff15a0128776 call dword ptr [shell32!_imp__RegOpenKeyExW (768712a0)]
Remember that function parameters are passed in in reverse order, so the second parameter is the second to last value passed to the function. In this case, we can see that it was stored in eax. Of course, eax has been overwritten by our return value of 2, but we can see that just before pushing eax, we get the value from ebp-0x270. Dumping that out as a Unicode string, we get the key we were looking for:
0:020> du @ebp-270h
14c5ed54 "Software\Microsoft\Windows\Curre"
14c5ed94 "ntVersion\Control Panel\Extended"
14c5edd4 " Properties\System.ApplicationNa"
14c5ee14 "me"
The first parameter is the root key, which was:
0:020> dc @ebp-278h L1
14c5ed4c 80000002
We can look up this value in the Windows SDK header files and see that it is for HKLM:
#define HKEY_LOCAL_MACHINE (( HKEY ) (ULONG_PTR)((LONG)0x80000002) )
Aha! So now we know we were trying to access “HKLM\ Software\Microsoft\Windows\CurrentVersion\Control Panel\Extended Properties\System.ApplicationName” which didn’t exist, so we were unable to proceed with loading the icon.
What’s that key for, you ask? MSDN tells us that it is used to store the Canonical Name of the Control Panel item. Canonical Names were added to the Control Panel in Windows Vista as a better way to organize and name the items in the Control Panel. By convention, the canonical name of “Mail” is “Microsoft.Office.Outlook.Profiles.” Because the “Show only specified control panel applets” policy can now accept either the legacy name (“Mail”) or the canonical name, we are checking the registry for this string. Since the key is not present, GetDetailsEx cannot determine the canonical name, and the icon does not load.
As a test, I created the key HKLM\ Software\Microsoft\Windows\CurrentVersion\Control Panel\Extended Properties\System.ApplicationName and added a REG_SZ to it with a name of the file location of the Mail CPL file (“C:\Program Files\Common Files\SYSTEM\MSMAPI\1033\MLCFG32.CPL”) and a value of “Microsoft.Office.Outlook.Profiles”. Sure enough, the icon loads up on the first try for new users.
While new Control Panel items should implement a canonical name, that doesn’t work for existing CPLs, like Mail from Outlook 2003. Since the canonical name isn’t actually required (CPL_DoesPolicyAllow works with both name formats), we’re following up with the development team to allow CPL_DoesPolicyAllow to be called even if a canonical name is not found.
-Matt Burrough
Expanding Netmon to aid in debugging
A walk-through of creating a Netmon parser in the context of a real case
This is an archive post of content I wrote for the NTDebugging Blog on MSDN. Since the MSDN blogs are being retired, I’m transferring my posts here so they aren’t lost. The post has been back-dated to its original publication date.
As is obvious to frequent readers of our blog, our team logs a lot of time in our debugger of choice (for some, windbg, for others, kd). However, a debugger is not always the best tool for the job, and sometimes even the best tool has limitations. I found this to especially true when working a few Internet Printing Protocol (IPP) cases recently.
Probably the biggest challenge of many IPP cases is the mixed environments you usually find IPP running in. The benefit customers see in IPP over other print providers is it works natively or with minimal configuration on Windows, Mac, and Linux clients. This makes it popular in places like college computer labs, where there isn’t one standard client system. Unfortunately, this also means that we can’t really debug both sides of the communication when something goes wrong.
In a recent case, a customer was having problems printing from their Windows Vista clients to a Linux Common Unix Printing System (CUPS) IPP server. If the CUPS server was set to allow anonymous connections, everything worked perfectly. When the administrator enabled authentication, he found that most print jobs failed to print. After a bit more testing, he found that small jobs (perhaps a page of printed text) worked fine, but larger, multi-page documents failed.
For situations like this, I prefer to use a network trace to get a feeling for where the problem is occurring. The problem was – IPP wasn’t one of the protocols built in to Netmon (and I find Wireshark’s IPP parser to not always work well – especially with Windows clients/servers). I decided that the amount of time it would take to decode the traffic by hand could be better spent creating a Netmon IPP parser that I could use every time I ran into one of these issues.
One of the great things about Netmon is you can view the source of every included parser. This was a big help, as I hadn’t written a parser before. [Note: all steps noted are written using Netmon 3.4.There might be slight differences in NM3.3.] To do this, open Netmon and click the Parsers tab. Under Object View, expand parser files and double click any of the .npl files. The source will appear on the right.
The language for Netmon parsers is similar to C++, with a limited set of statements. These are all documented in the Netmon help file, but the ones I found useful are described below. To begin, I started by defining a number of tables. The basic idea of a table is to provide a way to convert a value to a string. For example, one field in an IPP packet is the status of a printer, which is represented by an integer. In order to allow Netmon to show printer states in a readable form, I created a table to convert the values as seen in Figure 1 below.
Table IPPPrinterState //2911-4.4.11
{
switch(value)
{
case 3 : "idle";
case 4 : "processing";
case 5 : "stopped";
default : "Unknown Code";
}
}
Figure 1: Netmon Table
Each table is defined with the Table keyword, followed by a name for the table. (It may optionally be followed by a list of parameters, which I’ll use later. In this case, I added a comment that specified which RFC and section this information comes from.) A table consists of a switch statement with a case for each value, and a default for all other cases, much like other programming languages. I created tables like IPPPrinterState for each field that could be represented in an IPP packet from information I found in each of IPP’s RFCs.
Once the tables were complete, I moved on to creating the Protocol portion of the parser. This section of the code provides the logic that iterates through a packet and calls the tables for the appropriate data. This section starts with either the RegisterBefore or RegisterAfter keyword. These are used to determine how your parser is called. Essentially, Netmon takes all of the parsers it has, and compiles them into one continuous binary (.npb) and registration tells Netmon where your parser fits. For my case, I used the following registration code.
[ RegisterAfter (HTTPPayloadData.OCSP, Ipp, Property.HTTPContentType.Contains("application/ipp")) ]
This tells Netmon that, when compiling the parser, it should insert my code right after the code for the OCSP protocol in its HTTPPayloadData parser, my protocol should be called IPP, and it should enter my code path if the HTTP Payload is of content type “application/ipp”. This allows my parser to work a bit differently than the Wireshark IPP parser – Wireshark uses a port number (631) to identify IPP traffic, whereas my code looks at HTTP content types. The advantage of this, for me, is that Windows servers use port 80 for IPP by default, not 631, so in cases with a Windows IPP server, this parser should correctly identify the packets. You may be wondering how or why I chose to register after OCSP. Basically, I knew I needed my code to be registered in the section of code where HTTP does its payload content type processing. So I opened up HTTP’s parser, and searched for the content type analysis. OCSP was the first protocol I found in HTTP’s content type logic, so I used that as the place to insert my protocol.
After the registration comes the Protocol statement. I chose the following.
Protocol IPP = FormatString("Status/OpCode = %s", IPPOperationsSupported(OpID))
This names my protocol IPP and specifies that I want the description of the protocol to display the IPP status code. This way, a user doesn’t need to drill down to find out if this is a print job or a printer status request. You’ll notice FormatString is a function in Netmon that is similar to printf. In this case, I am passing a variable (OpID, which is defined lower in my code) to my IPPOperationsSupported table to determine what this OpCode means. Before I had a parser, I would need to look up the operations supported values in the IPP RFC for each packet I examined.
Next is the body of the protocol. Basically, this consists of a series of fields (like variables) that define how a packet is laid out. Creating a field is similar to declaring a variable in C++. You start by choosing a data type that matches the size of the data in the packet and provide a name for that field. For example, Figure 2 shown below contains the first seven lines of my Protocol.
struct Version = FormatString("%i.%i", Major, Minor)
{
INT8 Major;
INT8 Minor;
}
INT16 OpId = FormatString("%s (0x%X)", IPPOperationsSupported(this), this);
INT32 RequestId;
Figure 2: Code in the protocol block
The IPP specification states that all packets begin with two 8-bit values, the first value specifies the major protocol version in use, and the second value specifies the minor. In this case, I wrapped both in a struct so Netmon will display them as “Version: 1.0”, instead of separately as “Major: 1” “Minor: 0” on two lines. After the version is a 16-bit field that specifies the operation requested (for example, print-job or get-printer-state). I choose to display this value by looking it up in the IPPOperationsSupported table, then printing it as the string, followed by the hex value (e.g. “Get-Printer-Attributes (0xB)”). The ‘this’ keyword simply uses the value of the current field, which in this case is the OpId. Even though Netmon parses through the packet sequentially, this kind of use of a Field before its value is retrieved is allowed. Finally, I set the RequestId field, which is a 32-bit int value. Since this field is just a transaction ID for this conversation, I don’t need to do any formatting to it.
After that, things got a little more complicated. IPP’s structure allows for a variable number of attribute groups, each of which can contain a variable number of attributes. For example, in response to the request “Get-Printer-Attributes” from the client, the server responds with the Printer Attributes group, which contains a number of attributes like printer-state, queued-job-count, and so on. First, I needed to deal with the attribute groups in a loop until I’d read each one. IPP specifies that the end of the attribute groups is specified with the value of 0x03, so I wrote a while loop to create attribute groups until FrameData[FrameOffset] is equal to 3 (See Figure 3 below). FrameData and FrameOffset are special values provided by Netmon. FrameData is an array of the entire contents of the frame, and FrameOffset is Netmon’s current location in the packet. I use this instead of declaring a field here because referencing FrameData[FrameOffset] does not advance the parser frame location. This is important because I want to consume that value further down.
Inside that loop, I declared another struct that contains an attribute group. Much like the Protocol IPP line above, we reference a field here that will be declared lower down. This line does not advance the FrameOffset, since we don’t declare a field here. The first line of this struct is the field declaration line that finally consumes the attribute group tag. Below that is another While loop to process all attributes in the attribute group. IPP differentiates between attributes and attribute groups by making all attribute group identifiers smaller than 0x10, and all attribute identifiers 0x10 or higher. I use this as the condition for my loop. Finally, I declare an Attribute struct inside this loop. This struct is displayed after looking up how to properly print based on the Attribute Name and Value in the AttribDisplayTable.
IPP declares attributes as an 8-bit type identifier (int, bool, string, etc.), a 16-bit int specifying the attribute name’s length, the name (a string), a 16-bit in value length, and a value. Since I want to look up the value in various tables, depending on the Attribute Name, I store the Attribute Name as “AttName” in a property. This way, I can continue to reference it while processing continues. Properties are declared in brackets just above the field they will store. In my case, I prepend the ‘Post.’ evaluation keyword to the property name. This instructs Netmon to use the end result of the next line as its value, but before advancing the FrameOffset. I do this again for the actual value, which I call Val. If I did not use the Post evaluation keyword, Val would contain the unsigned int32 value of printer state, instead of the formatted string result I get by looking up printer state in its table.
While [FrameData[FrameOffset] != 0x03]
{
struct AttributeGroup = FormatString("%s", IPPTags(TagGroup))
{
INT8 TagGroup = FormatString("%s (0x%X)", IPPTags(this), this);
While [FrameData[FrameOffset] >= 0x10]
{
struct Attribute = AttribDisplayTable(AttName, Val)
{
INT8 Type = FormatString("%s (0x%X)", IPPTags(this), this);
INT16 NameLength;
[Post.AttName]
AsciiString(NameLength) AttributeName;
INT16 ValueLength;
switch(AttName)
{
case "printer-state" :
[Post.Val]
UINT32 PrinterState = FormatString("%s (0x%X)", IPPPrinterState(this),this);
...
Figure 3: Loops in protocol block
My case statements continue like printer-state for all possible attributes of IPP. At the very end of the protocol block, after I’ve closed my switch, structs, and whiles, I have one more line, which consumes any data remaining in the packet. This would contain document data if the packet was a print job, and is required so all the packet data is consumed before Netmon moves on to the next packet. That line is:
BLOB (FrameLength – FrameOffset) Data;
As you can see, it is a binary blob data type, set to the size of the frame, less our current location.
Finally, after my Protocol block, I needed to define my own data type. IPP defines its own data format to specify printer uptime, so I created a data type for it as shown below in Figure 4.
//Uptime format spec
Number UPTIME
{
Size = 4;
DisplayFormat = (this != 0) ? FormatString("%i days %02i:%02i:%02i (%i seconds)",
(this/86400), //Days
(this-((this/86400)*86400))/3600, //Hours
(this-(((this/86400)*86400)+(((this-((this/86400)*86400))/3600)*3600)))/60, //Minutes
(this%60), //Seconds
this) : "0"
}
Figure 4: Custom data type
The first line of Figure 4 specifies this will be a data type composed of numeric data named UPTIME. Size specifies how many bytes the type uses. DisplayFormat is what Netmon displays for this type. In this case, I use the x ? y : z syntax. Netmon doesn’t have if/then/else keywords, but instead uses this ternary operator. I use a special case for 0 since it seems to be a common return value in the traces I’ve looked at, and having ‘Uptime: 0 days 00:00:00 (0 seconds)’ seemed excessive.
Figures 5 and 6 below show what the result looks like in Netmon.
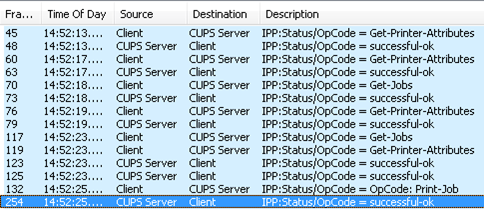
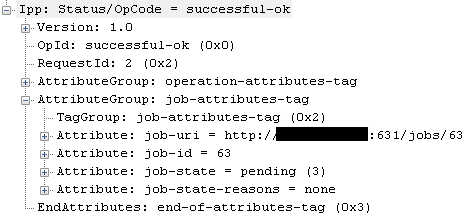
So what did the trace show? Windows attempts to send IPP requests with no authentication first, then if it receives an access denied, retries with authentication. This is by design, as the IPP server replies with the authentication types it supports in the access denied message. In the case of print jobs that are too large to fit in a single packet, IPP’s spec allows servers to either issue the access denied message as soon as it receives the first packet, or after it has received the entire job. It turns out that the IPP Print Provider on Windows was designed to send the entire job before listening for a response, so it missed the access denied message that CUPS sent after it received the first packet. See http://support.microsoft.com/kb/976988/ [New link https://support.microsoft.com/en-us/topic/you-may-be-unable-to-print-to-an-ipp-print-queue-in-windows-7-or-in-windows-server-2008-r2-44a0f58a-4894-f98c-4a82-ed66eeb84ded] for related information. Want a copy of the IPP parser? It will be included in a future release of the Netmon Parser Update [Archived at https://codeplexarchive.org/project/NMParsers, code also shown below].
I hope this post have given you a better idea of how Netmon works, how IPP works, and helps if you ever need to write a parser for your protocol.
-Matt Burrough
//# (c) 2010 Outercurve Foundation
//#
//# Title: Internet Printing Protocol v1.1
//#
//# Details:
//#
//# Public References: RFC 2910, 2911, 3380, 3381, 3382, 3995, 3996, 3998, pwg5100.1, pwg5100.5, pwg5100.7, pwg5100.8
//#
//# Comments:
//#
//# Revision Class and Date:Major, 07/28/2011
//#
//####
[ RegisterAfter(HTTPPayloadData.OCSP, IPP, Property.HTTPContentType.Contains("application/ipp")) ]
Protocol IPP = FormatString(Property.HttpIsRequest == 0x1 ? "OperationID = " + IPPOperationsID(OperationID):"StatusCode = " + IPPStatusCodes(StatusCode))
{
[ MaxLoopCount = 1 ]
while [ FrameData[ FrameOffset ]==0x01 && FrameData[ FrameOffset + 1 ] <= 0x01 ] // Only support IPP v1.0/1.1 (latest version released)
{
struct Version = FormatString("%i.%i", Major, Minor)
{
INT8 Major;
INT8 Minor;
}
switch
{
case Property.HttpIsRequest == 0x1:
UINT16 OperationID = FormatString("%s (0x%X)", IPPOperationsID(this), this);
case Property.HttpIsResponse == 0x1:
UINT16 StatusCode = FormatString("%s (0x%X)", IPPStatusCodes(this), this);
default:
ReportParserError(ParserErrorProtocolClassWindows, "IPP", "Unknown Message" ) MessageError;
}
INT32 RequestId;
While [ FrameData [ FrameOffset ] != 0x03 ]
{
struct AttributeGroup = FormatString("%s", IPPTags(TagGroup))
{
INT8 TagGroup = FormatString("%s (0x%X)", IPPTags(this), this);
While [FrameData[FrameOffset] >= 0x10]
{
struct Attribute = FormatString("%s %s %s", AttName, DrawEquals(ValueLength), AttribDisplayTable(AttName, Ret))
{
INT8 Type = FormatString("%s (0x%X)", IPPTags(this), this);
INT16 NameLength;
[Post.AttName]
AsciiString(NameLength) AttributeName;
INT16 ValueLength;
[Post.Val]
ValueStr(AttName, Type, ValueLength) Value = AttribDisplayTable(AttName, Ret);
while [FrameData[FrameOffset + 1] == 0 && FrameData[FrameOffset + 2] == 0]
{
struct AdditionalAttribute = AdditionalValue.ToString
{
INT8 AdditionalType = FormatString("%s (0x%X)", IPPTags(this), this);
INT16 AdditionalNameLength;
INT16 AdditionalValueLength;
[PostAfter.Ret = "..."]
ValueStr(AttName, AdditionalType, AdditionalValueLength) AdditionalValue = AttribDisplayTable(AttName, Ret);
}
}
}
}
}
}
INT8 EndAttributes = FormatString("%s (0x%X)", IPPTags(this), this); // end-of-attribues tag
}
BLOB (FrameLength - FrameOffset) Data; // For things like print job binary data
}
// Tables of value codes from IPP RFCs
Table IPPTags(value) //2910-3.5
{
switch(value)
{
// Delimiter Tags
case 0x00 : "reserved";
case 0x01 : "operation-attributes-tag";
case 0x02 : "job-attributes-tag";
case 0x03 : "end-of-attributes-tag";
case 0x04 : "printer-attributes-tag";
case 0x05 : "unsupported-attributes-tag";
case 0x06 : "subscription-attributes-tag";
case 0x07 : "event-notification-attributes-tag";
case 0x09 : "document-attributes-tag"; // pwg5100.5
// Value Tags
case 0x10 : "unsupported";
case 0x11 : "reserved";
case 0x12 : "unknown";
case 0x13 : "no-value";
case 0x15 : "not-settable"; // 3380
case 0x16 : "delete-attribute";
case 0x17 : "admin-define";
// Types
case 0x20 : "reserved-generic-integer";
case 0x21 : "integer";
case 0x22 : "boolean";
case 0x23 : "enum";
// OctectString
case 0x30 : "octetString with an unspecified format";
case 0x31 : "dateTime";
case 0x32 : "resolution";
case 0x33 : "rangeOfInteger";
case 0x34 : "begCollection";
case 0x35 : "textWithLanguage";
case 0x36 : "nameWithLanguage";
case 0x37 : "endCollection";
// Character Strings
case 0x40 : "reserved-generic-char-str";
case 0x41 : "textWithoutLanguage";
case 0x42 : "nameWithoutLanguage";
case 0x43 : "reserved";
case 0x44 : "keyword";
case 0x45 : "uri";
case 0x46 : "uriScheme";
case 0x47 : "charset";
case 0x48 : "naturalLanguage";
case 0x49 : "mimeMediaType";
case 0x4A : "memberAttrName"; // 3382
case 0x7F : "Extension";
default : "Unknown or Reserved Tag";
}
}
Table IPPFinishing(value) // 2911-4.2.6
{
switch(value)
{
case 3 : "none";
case 4 : "staple";
case 5 : "punch";
case 6 : "cover";
case 7 : "bind";
case 8 : "saddle-stitch";
case 9 : "edge-stitch";
case 10 : "fold"; // pwg5100.1
case 11 : "trim";
case 12 : "blae";
case 13 : "booklet-maker";
case 14 : "jog-offset";
case 20 : "staple-top-left";
case 21 : "staple-bottom-left";
case 22 : "staple-top-right";
case 23 : "staple-bottom-right";
case 24 : "edge-stitch-left";
case 25 : "edge-stitch-top";
case 26 : "edge-stitch-right";
case 27 : "edge-stitch-bottom";
case 28 : "staple-dual-left";
case 29 : "staple-dual-top";
case 30 : "staple-dual-right";
case 31 : "staple-dual-bottom";
case 50 : "bind-left";
case 51 : "bind-top";
case 52 : "bind-right";
case 53 : "bind-bottom";
default : "Unknown Code";
}
}
Table IPPOrientationRequested(value) // 2911-4.2.10
{
switch(value)
{
case 3 : "portrait";
case 4 : "landscape";
case 5 : "reverse-landscape";
case 6 : "reverse-portrait";
default : "Unknown Code";
}
}
Table IPPPrintQuality(value) // 2911-4.2.13
{
switch(value)
{
case 3 : "draft";
case 4 : "normal";
case 5 : "high";
default : "Unknown Code";
}
}
Table IPPJobCollation(value) // 3381-4.1
{
switch(value)
{
case 1 : "Other";
case 2 : "Unknown";
case 3 : "Uncollated-Sheets";
case 4 : "Collated-Documents";
case 5 : "Uncollated-Documents";
default : "Unknown Code";
}
}
Table IPPJobState(value) // 2911-4.3.7
{
switch(value)
{
case 3 : "pending";
case 4 : "pending-held";
case 5 : "processing";
case 6 : "processing-stopped";
case 7 : "canceled";
case 8 : "aborted";
case 9 : "completed";
default : "Unknown Code";
}
}
Table IPPDocumentState(value) // pwg5100.5
{
switch(value)
{
case 3 : "Pending";
case 5 : "Processing";
case 7 : "Canceled";
case 8 : "Aborted";
case 9 : "Completed";
default : "Unknown State";
}
}
Table IPPPrinterState(value) // 2911-4.4.11
{
switch(value)
{
case 3 : "idle";
case 4 : "processing";
case 5 : "stopped";
default : "Unknown Code";
}
}
Table IPPUnits(value) // 2911-4.1.15
{
switch(value)
{
case 3 : "dpi";
case 4 : "dpcm";
}
}
Table IPPOperationsID(value) // 2911-4.4.15
{
switch(value)
{
// Op Codes
case 0x0000 : "reserved";
case 0x0001 : "reserved";
case 0x0002 : "Print-Job";
case 0x0003 : "Print-URI";
case 0x0004 : "Validate-Job";
case 0x0005 : "Create-Job";
case 0x0006 : "Send-Document";
case 0x0007 : "Send-URI";
case 0x0008 : "Cancel-Job";
case 0x0009 : "Get-Job-Attributes";
case 0x000A : "Get-Jobs";
case 0x000B : "Get-Printer-Attributes";
case 0x000C : "Hold-Job";
case 0x000D : "Release-Job";
case 0x000E : "Restart-Job";
case 0x000F : "reserved";
case 0x0010 : "Pause-Printer";
case 0x0011 : "Resume-Printer";
case 0x0012 : "Purge-Jobs";
case 0x0013 : "Set-Printer-Attributes"; // 3380
case 0x0014 : "Set-Job-Attributes";
case 0x0015 : "Get-Printer-Supported-Values";
case 0x0016 : "Create-Pritner-Subscriptions"; // 3995
case 0x0017 : "Create-Job-Subscriptions";
case 0x0018 : "Get-Subscription-Attributes";
case 0x0019 : "Get-Subscriptions";
case 0x001A : "Renew-Subscription";
case 0x001B : "Cancel-Subscription";
case 0x001C : "Get-Notifications"; // 3996
case 0x0021 : "Get-Client-Printer-Support-Files"; // http://www.ietf.org/proceedings/48/I-D/ipp-install-00.txt
case 0x0022 : "Enable-Printer"; // 3998
case 0x0023 : "Disable-Printer";
case 0x0024 : "Pause-Printer-After-Current-Job";
case 0x0025 : "Hold-New-Jobs";
case 0x0026 : "Release-Held-New-Jobs";
case 0x0027 : "Deactivate-Printer";
case 0x0028 : "Activate-Printer";
case 0x0029 : "Restart-Printer";
case 0x002A : "Shutdown-Printer";
case 0x002B : "Startup-Printer";
case 0x002C : "Reprocess-Job";
case 0x002D : "Cancel-Current-Job";
case 0x002E : "Suspend-Current-Job";
case 0x002F : "Resume-Job";
case 0x0030 : "Promote-Job";
case 0x0031 : "Schedule-Job-After";
case 0x0033 : "Cancel-Document"; // pwg5100.5
case 0x0034 : "Get-Document-Attributes";
case 0x0035 : "Get-Documents";
case 0x0036 : "Delete-Document";
case 0x0037 : "Set-Document-Attributes";
// Vendor-Specific codes (0x4000-0xFFFF) (http://www.pwg.org/ipp/opcodes/ippopcodes.html)
case 0x4000 : "Microsoft Specific Auth";
case 0x4001 : "CUPS-Get-Default"; // http://www.cups.org/documentation.php/spec-ipp.html
case 0x4002 : "CUPS-Get-Printers";
case 0x4003 : "CUPS-Add-Modify-Printer";
case 0x4004 : "CUPS-Delete-Printer";
case 0x4005 : "CUPS-Get-Classes";
case 0x4006 : "CUPS-Add-Modify-Class";
case 0x4007 : "CUPS-Delete-Class";
case 0x4008 : "CUPS-Accept-Jobs";
case 0x4009 : "CUPS-Reject-Jobs";
case 0x400A : "CUPS-Set-Default";
case 0x400B : "CUPS-Get-Devices";
case 0x400C : "CUPS-Get-PPDs";
case 0x400D : "CUPS-Move-Job";
case 0x400E : "CUPS-Authenticate-Job";
case 0x400F : "CUPS-Get-PPD";
case 0x4010 : "Peerless Systems Specific";
case 0x401A : "novell-list-printers"; // https://www1.ietf.org/mail-archive/text/ipp/2008-03.mail
case 0x401B : "novell-list-drivers";
case 0x401C : "novell-mgmt-interface";
case 0x401D : "novell-resource-add";
case 0x401E : "novell-resource-list";
case 0x401F : "novell-resource-delete";
case 0x4020 : "novell-resource-download";
case 0x4021 : "novell-get-driver-profile";
case 0x4022 :
case 0x4023 :
case 0x4024 :
case 0x4025 : "Novell Specific";
case 0x4026 : "Xerox Specifc";
case 0x4027 : "CUPS-Get-Document";
default : "Unknown Operations ID";
}
}
Table IPPStatusCodes(value) // 2911-4.4.15
{
switch
{
// Status Codes
// 0x0000:0x00FF - "successful"
case value == 0x0000 : "successful-ok";
case value == 0x0001 : "successful-ok-ignored-or-substituted-attributes";
case value == 0x0002 : "successful-ok-conflicting-attributes";
case value == 0x0003 : "successful-ok-ignored-subscriptions";
case value == 0x0005 : "successful-ok-too-many-events";
case value == 0x0007 : "successful-ok-events-complete";
case value >= 0x0100 && value <= 0x01FF: "informational";
case value >= 0x0300 && value <= 0x03FF: "redirection";
// 0x0400:0x04FF - "client-error"
case value == 0x0400 : "client-error-bad-request";
case value == 0x0401 : "client-error-forbidden";
case value == 0x0402 : "client-error-not-authenticated";
case value == 0x0403 : "client-error-not-authorized";
case value == 0x0404 : "client-error-not-possible";
case value == 0x0405 : "client-error-timeout";
case value == 0x0406 : "client-error-not-found";
case value == 0x0407 : "client-error-gone";
case value == 0x0408 : "client-error-request-entity-too-large";
case value == 0x0409 : "client-error-request-value-too-long";
case value == 0x040A : "client-error-document-format-not-supported";
case value == 0x040B : "client-error-attributes-or-values-not-supported";
case value == 0x040C : "client-error-uri-scheme-not-supported";
case value == 0x040D : "client-error-charset-not-supported";
case value == 0x040E : "client-error-conflicting-attributes";
case value == 0x040F : "client-error-compression-not-supported";
case value == 0x0410 : "client-error-compression-error";
case value == 0x0411 : "client-error-document-format-error";
case value == 0x0412 : "client-error-document-access-error";
case value == 0x0413 : "client-error-attributes-not-settable"; // 3380
case value == 0x0414 : "client-error-ignored-all-subscriptions"; // 3995
case value == 0x0415 : "client-error-too-many-subscriptions";
case value == 0x0417 : "clnt-err-client-print-support-file-not-found"; // http://www.ietf.org/proceedings/48/I-D/ipp-install-00.txt
//0x0500:0x05FF - "server-error"
case value == 0x0500 : "server-error-internal-error";
case value == 0x0501 : "server-error-operation-not-supported";
case value == 0x0502 : "server-error-service-unavailable";
case value == 0x0503 : "server-error-version-not-supported";
case value == 0x0504 : "server-error-device-error";
case value == 0x0505 : "server-error-temporary-error";
case value == 0x0506 : "server-error-not-accepting-jobs";
case value == 0x0507 : "server-error-busy";
case value == 0x0508 : "server-error-job-canceled";
case value == 0x0509 : "server-error-multiple-document-jobs-not-supported";
case value == 0x050A : "server-error-printer-is-deactivated"; // 3398
case value == 0x050B : "server-error-too-many-jobs"; // pwg5100.7
case value == 0x050C : "server-error-too-many-documents";
default : "Unknown Status Code";
}
}
Table DrawEquals(ValLen)
{
switch(ValLen)
{
case 0 : "";
default : "=";
}
}
// Tables for displaying attribute summaries
Table AttribDisplayTable(Attrib, Val)
{
switch(val)
{
case "..." : // Don't try to format multi-value attributes
FormatString("%s", Val);
default:
AttribFormatTable(Attrib, Val);
}
}
Table AttribFormatTable(Attrib, Val)
{
switch(Attrib)
{
case "printer-state" :
FormatString("%s (%u)", IPPPrinterState(Val),Val);
case "finishings" :
case "finishings-actual" : // pwg5100.8
FormatString("%s (%u)", IPPFinishing(Val),Val);
case "orientation-requested" :
case "orientation-requested-actual" : // pwg5100.8
FormatString("%s (%u)", IPPOrientationRequested(Val),Val);
case "print-quality" :
case "print-quality-actual" : // pwg5100.8
FormatString("%s (%u)", IPPPrintQuality(Val),Val);
case "job-state" :
FormatString("%s (%u)", IPPJobState(Val),Val);
case "job-collation-type" : // 3381
FormatString("%s (%u)", IPPJobCollation(Val),Val);
case "document-state" :
FormatString("%s (%u)", IPPDocumentState(Val),Val);
case "operations-supported" :
FormatString("%s (%u)", IPPOperationsID(Val),Val);
case "printer-is-accepting-jobs" :
case "multiple-document-jobs-supported" :
case "color-supported" :
(Val!=0) ? FormatString("TRUE (0x01)") : FormatString("FALSE (0x00)");
case "time-at-creation" :
case "time-at-processing" :
case "time-at-completed" :
case "job-printer-up-time" :
case "printer-up-time" :
FormatString("%i days %02i:%02i:%02i (%i seconds)",
(Val/86400), // Days
(Val-((Val/86400)*86400))/3600, // Hours
(Val-(((Val/86400)*86400)+(((Val-((Val/86400)*86400))/3600)*3600)))/60, // Minutes
(Val%60),
Val);
default :
FormatString("%s", Val);
}
}
// Uptime format spec
Number UPTIME
{
Size = 4;
DisplayFormat = FormatString("%i days %02i:%02i:%02i (%i seconds)",
(this / 86400), // Days
(this - ((this / 86400) * 86400)) / 3600, // Hours
(this - (((this / 86400) * 86400) + (((this - ((this / 86400) * 86400)) / 3600) * 3600))) / 60, // Minutes
(this % 60), // Seconds
this) // Total secs
}
struct ValueStr(AttName, Type, ValueLength) = Ret
{
switch(AttName)
{
case "printer-state" :
[Ret]
UINT32 PrinterState = FormatString("%s (%u)", IPPPrinterState(this),this);
case "finishings" :
case "finishings-actual" : // pwg5100.8
[Ret]
UINT32 Finishings = FormatString("%s (%u)", IPPFinishing(this),this);
case "orientation-requested" :
case "orientation-requested-actual" : // pwg5100.8
[Ret]
UINT32 Orientation = FormatString("%s (%u)", IPPOrientationRequested(this),this);
case "print-quality" :
case "print-quality-actual" : // pwg5100.8
[Ret]
UINT32 PrintQuality = FormatString("%s (%u)", IPPPrintQuality(this),this);
case "job-state" :
[Ret]
UINT32 JobState = FormatString("%s (%u)", IPPJobState(this),this);
case "job-collation-type" : // 3381
[Ret]
UINT32 JobCollation = FormatString("%s (%u)", IPPJobCollation(this),this);
case "document-state" :
[Ret]
UINT32 DocumentState = FormatString("%s (%u)", IPPDocumentState(this),this);
case "operations-supported" :
[Ret]
UINT32 OperationsSupported = FormatString("%s (%u)", IPPOperationsID(this),this);
case "time-at-creation" :
case "time-at-processing" :
case "time-at-completed" :
case "job-printer-up-time" :
case "printer-up-time" :
[Ret]
UPTIME TimeValue;
default :
switch(Type)
{
case 0x20 :
[Ret]
INT32 ReservedIntValue;
case 0x21 :
[Ret]
INT32 IntValue;
case 0x22 :
[Ret]
BOOLEAN BoolValue;
case 0x23 :
[Ret]
UINT32 EnumValue;
// OctectString
case 0x31 : //2911 4.1.14 --> RFC1903
[Ret]
struct DateAndTime = FormatString("%u-%u-%u,%u:%u:%u.%u,%c%u:%u", Year, Month, Day, Hour, Minute, Second, DeciSec, DUTC, HUTC, MUTC)
{
// DISPLAY-HINT "2d-1d-1d,1d:1d:1d.1d,1a1d:1d"
// field octets contents range
// ----- ------ -------- -----
UINT16 Year; // 1 1-2 year* 0..65536
UINT8 Month; // 2 3 month 1..12
UINT8 Day; // 3 4 day 1..31
UINT8 Hour; // 4 5 hour 0..23
UINT8 Minute; // 5 6 minutes 0..59
UINT8 Second; // 6 7 seconds 0..60 (use 60 for leap-second)
UINT8 DeciSec; // 7 8 deci-seconds 0..9
CHAR DUTC; // 8 9 direction from UTC '+' / '-'
UINT8 HUTC; // 9 10 hours from UTC* 0..13
UINT8 MUTC; // 10 11 minutes from UTC 0..59
// For example, Tuesday May 26, 1992 at 1:30:15 PM EDT would be
// 1992-5-26,13:30:15.0,-4:0
}
case 0x32 : //2911 4.1.15 "resolution" --> RFC1759
[Ret]
struct Resolution = FormatString("%ix%i %s",CrossFeedRes, FeedRes, IPPUnits(Units))
{
INT32 CrossFeedRes;
INT32 FeedRes;
INT8 Units;
}
case 0x33 : // "rangeOfInteger"
[Ret]
struct Range = FormatString("%i-%i",lower, upper)
{
INT32 lower;
INT64 upper;
}
default:
[Ret]
AsciiString(ValueLength) StringValue;
}
}
}
Case Study – Software Restriction Policies and Large EXE Files
This is an archive post of content I wrote for the NTDebugging Blog on MSDN. Since the MSDN blogs are being retired, I’m transferring my posts here so they aren’t lost. The post has been back-dated to its original publication date.
Recently I received a debug request for a customer having problems running large executables. On their systems, they could run most EXEs without any problems, but they had two programs that were over 1.8 GB which, when run, would display the following error:

If they tried to run them in a command prompt, they received the message “Access is denied.” Both attempts were made with an administrator account and in neither case were the processes created. Through testing, they found that the programs worked if they were scheduled to run as System and also worked when run in safe mode as an administrator.
When the case was brought to my attention, it was noted that when the failing executables were run, the following appeared in process monitor logs:

The engineer did not see this when one of the problematic EXEs was run (successfully) on his test machine. The customer provided a VM image of their system which we set up in HyperV with a named pipe kernel debugger. I then started kernel debugging to find the cause of the INVALID PARAMETER error, hoping that resolving it would fix the issue.
To start, I looked at the call stack within process monitor for the invalid parameter:
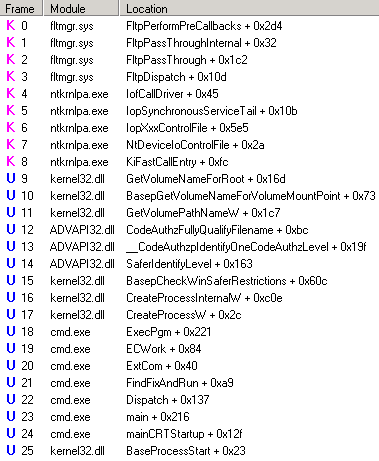
The problem is this isn’t exactly where we return invalid parameter. Looking at the source code for Fltmgr, it doesn’t return invalid parameter – this was just where the error was caught in procmon. This call stack did provide some ideas for good starting places to debug, however. First, I looked up the hex value for STATUS_INVALID_PARAMETER in ntstatus.h – 0xC000000D. Knowing this, I decided to set a breakpoint on nt!IofCallDriver and ran the program. Once the debugger broke in, I planned to use wt -oR. This will trace through the calls displaying the return values next to each call. From there, I would just need to find 0xc000000d on the return column. Unfortunately, I had forgotten was wt does not display return codes in kernel debugging, only when debugging user mode.
With wt not an option, I decided to use a combination of debugger commands to approximate the output of wt. I knew the return value I was looking for, and I was also confident that I would find that code in the EAX register after the problem occurred. As such, I needed to write a loop that would walk through the instructions until it found 0xC000000D in EAX. The debugger provides two main options for walking instructions: p and t. p (Step) will execute a single instruction and display the register values. If the instruction is a call, it will not enter that function, but just display the results after that subroutine has been executed. t (Trace) also executes a single instruction, but it will enter into the function and will display each instruction.
In this case I wanted trace so I could see which function was returning the invalid parameter status. Tracing though that many instructions/functions would take a long time, but there are some variations on t (and p) that can help. tc (or pc)will execute instructions until a call statement is reached, where it will break and show the register values. tt (or pt) will execute instructions until a return instruction is reached. tct (or pct) will run until either a call or return is reached. In this case, I opted for tct.
Knowing that I would use tct, I had to find a way to execute tct statements until EAX was the value I was looking for. This can be accomplished with the z (While) debugger command. The syntax is pretty easy, it’s just z(expression) and it works just like a do-while loop. Putting it all together, I used this command in the debugger:
tct; z(eax!=0xc000000d)
I then waited for the debugger to break in so I could see where this status was being thrown. Regrettably, the code called ended up going in to some recursion which made my while loop take way too long. To resolve this, I set a new breakpoint just before we entered the recursion, reran the program, used p to step past the call then ran the tct loop.
This time I was quickly brought to the code I was looking for. As soon as it broke in, I ran k to view the callstack:
kd> k
ChildEBP RetAddr
b9541a3c f7b7fab9 Ntfs!NtfsCommonDeviceControl+0x40
b9541aa0 f7b8b02f Ntfs!NtfsFsdDispatchSwitch+0xe4
b9541bbc 8081df85 Ntfs!NtfsFsdDispatchWait+0x1c
b9541bd0 f7876d28 nt!IofCallDriver+0x45
b9541bfc 8081df85 fltmgr!FltpDispatch+0x152
b9541c10 f7876d28 nt!IofCallDriver+0x45
b9541c3c 8081df85 fltmgr!FltpDispatch+0x152
b9541c50 808f5437 nt!IofCallDriver+0x45
b9541c64 808f61bf nt!IopSynchronousServiceTail+0x10b
b9541d00 808eed08 nt!IopXxxControlFile+0x5e5
b9541d34 808897bc nt!NtDeviceIoControlFile+0x2a
b9541d34 7c82860c nt!KiFastCallEntry+0xfc
0012e960 7c826fe9 ntdll!KiFastSystemCallRet
0012e964 77e416f9 ntdll!NtDeviceIoControlFile+0xc
0012e9c8 77e6738d kernel32!DeviceIoControl+0x137
0012ec44 77e67032 kernel32!GetVolumeNameForRoot+0x16d
0012ec68 77e67782 kernel32!BasepGetVolumeNameForVolumeMountPoint+0x73
0012ecd0 7d20b01d kernel32!GetVolumePathNameW+0x1c7
0012ef18 7d20ae2c ADVAPI32!CodeAuthzFullyQualifyFilename+0xbc
0012eff8 7d20b33f ADVAPI32!__CodeAuthzpIdentifyOneCodeAuthzLevel+0x19f
0012f07c 77e6df9e ADVAPI32!SaferIdentifyLevel+0x163
0012f278 77e6ce03 kernel32!BasepCheckWinSaferRestrictions+0x60c
0012fa90 77e424b0 kernel32!CreateProcessInternalW+0xc0e
0012fac8 4ad0256f kernel32!CreateProcessW+0x2c
0012fc24 4ad01a2b cmd!ExecPgm+0x221
0012fc58 4ad019b3 cmd!ECWork+0x84
0012fc70 4ad03c58 cmd!ExtCom+0x40
0012fe9c 4ad01447 cmd!FindFixAndRun+0xa9
0012fee0 4ad0c30b cmd!Dispatch+0x137
0012ff44 4ad07786 cmd!main+0x216
0012ffc0 77e6f23b cmd!mainCRTStartup+0x12f
0012fff0 00000000 kernel32!BaseProcessStart+0x23
If we look at the assembly around Ntfs!NtfsCommonDeviceControl+0x40, we see that mov 0xC000000D in to esi, then mov esi to eax if our return from NtfsDecodeFileObject isn’t 4:
f7b7faf9 e8e904fdff call Ntfs!NtfsDecodeFileObject (f7b4ffe7)
f7b7fafe 83f804 cmp eax,4
f7b7fb01 0f848873ffff je Ntfs!NtfsCommonDeviceControl+0x54 (f7b76e8f)
Ntfs!NtfsCommonDeviceControl+0x40:
f7b7fafe 83f804 cmp eax,4
f7b7fb01 0f848873ffff je Ntfs!NtfsCommonDeviceControl+0x54 (f7b76e8f)
f7b7fb07 be0d0000c0 mov esi,0C000000Dh
f7b7fb0c 56 push esi
f7b7fb0d 53 push ebx
f7b7fb0e 57 push edi
f7b7fb0f e83506fdff call Ntfs!NtfsCompleteRequest (f7b50149)
f7b7fb14 8bc6 mov eax,esi
I looked at the source code for these functions, and it didn’t make sense that a failure here would cause the problems we were seeing; especially specific to large executables. Out of curiosity I ran notepad on the VM while logging in perfmon and found that it too displayed INVALID PARAMETER, but the program stated and ran correctly:

Since this wasn’t the problem, I stopped reviewing the code and decided on a new approach. We knew that when running the EXE in a command prompt we received an Access is denied message. At that point it made sense to switch to user mode debugging and take a look at the cmd.exe process that was trying to launch install.exe.
Doing user mode debugging in a VM can be a bit of a challenge, especially if you are trying to minimize changes to the VM (and in my case, avoid putting any symbols on the customer’s VM image). Since I already had a kernel debugger attached, one option would be to run ntsd.exe (debugger provided in the Debugging Tools for Windows) on the VM with the -p switch specifying the PID of the cmd.exe process I wanted to debug and -d switch which forwards the i/o of ntsd to the kernel debugger. The problem with this approach is the kernel debugger just becomes a front end for issuing commands and seeing the output from ntsd. That means all symbol resolution is still done on the target system running ntsd.
I didn’t want give the customer VM Internet or corporate network access, so I instead opted to run dbgsrv.exe on the VM. Running “dbgsrv -t tcp:port=9999” tells the debug server to listen on TCP port 9999 for debugger connections. Then, on the HyperV server computer I could just run windbg -premote tcp:server=(IP of VM),port=9999 -p (PID of cmd on VM) to debug it.
I suspected that we may be calling CreateProcess but it was failing, so I set a breakpoint kernel32!CreateProcessW. Sure enough, it hit when I tried to run install.exe in the command prompt:
0:000> k
ChildEBP RetAddr
0012fac8 4ad0256f kernel32!CreateProcessW
0012fc24 4ad01a2b cmd!ExecPgm+0x221
0012fc58 4ad019b3 cmd!ECWork+0x84
0012fc70 4ad03c58 cmd!ExtCom+0x40
0012fe9c 4ad01447 cmd!FindFixAndRun+0xa9
0012fee0 4ad0c30b cmd!Dispatch+0x137
0012ff44 4ad07786 cmd!main+0x216
0012ffc0 77e6f23b cmd!mainCRTStartup+0x12f
0012fff0 00000000 kernel32!BaseProcessStart+0x23
This time I could use wt -oR since this was a usermode debug. Looking in ntstatus.h again, the code for STATUS_ACCESS_DENIED is 0xC0000022. Running wt can take a very long time, so I used the -l switch, which limits the number of levels deep it will display. This would be something like using tct as I did above until you were a few calls deep then using pct. Using wt -l 3 -oR gave me the following:
…
575 291 [ 1] kernel32!CreateProcessInternalW
35 0 [ 2] kernel32!BasepCheckWinSaferRestrictions
25 0 [ 3] ntdll!RtlEnterCriticalSection eax = 0
48 25 [ 2] kernel32!BasepCheckWinSaferRestrictions
1 0 [ 3] ntdll!NtOpenThreadToken
3 0 [ 3] ntdll!ZwOpenThreadToken eax = ffffffff`c000007c
57 29 [ 2] kernel32!BasepCheckWinSaferRestrictions
1 0 [ 3] ntdll!ZwOpenProcessToken
3 0 [ 3] ntdll!NtOpenProcessToken eax = 0
116 33 [ 2] kernel32!BasepCheckWinSaferRestrictions
113 0 [ 3] ADVAPI32!SaferIdentifyLevel eax = 0
130 146 [ 2] kernel32!BasepCheckWinSaferRestrictions
4 0 [ 3] ntdll!ZwClose eax = 0
132 150 [ 2] kernel32!BasepCheckWinSaferRestrictions
22 0 [ 3] ntdll!RtlLeaveCriticalSection eax = 0
138 172 [ 2] kernel32!BasepCheckWinSaferRestrictions
5 0 [ 3] kernel32!__security_check_cookie eax = ffffffff`c0000022
…
Now we are getting close! I set a new breakpoint for kernel32!BasepCheckWinSaferRestrictions and reran the test. This gave me the following line:
63 0 [ 3] ADVAPI32!__CodeAuthzpCheckIdentityHashRules eax = ffffffff`c0000022
One last run with a new breakpoint at ADVAPI32!__CodeAuthzpCheckIdentityHashRules and I found what I was looking for:
58 218 [ 1] ADVAPI32!__CodeAuthzpEnsureMapped eax = ffffffff`c0000022
The depth is shown in brackets. As this call was 1 deep from __CodeAuthzpCheckIdentityHashRules and I was using 3 as my maximum depth in wt, I knew this is where the access denied was coming from. I reviewed the source code and found that this is the code that performs Software Restriction Policy checking. Specifically, we are attempting to map the executable into memory to perform hash checking on it. Since there isn’t 1.8 GB of contiguous available memory, it failed. Looking at the VM, I discovered that the customer had implemented a number of software restriction policies. As a test, I removed their restrictions on the VM, and the programs ran successfully. A search of the KB revealed that a hotfix was published for this problem: 973825. Installing the hotfix in the article also resolved the issue with their policies intact.
-Matt Burrough